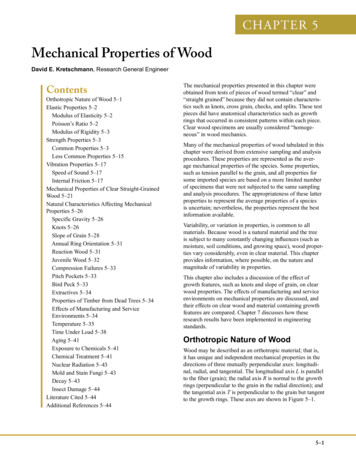
Transcription
CHAPTER 5Mechanical Properties of WoodDavid E. Kretschmann, Research General EngineerContentsOrthotropic Nature of Wood 5–1Elastic Properties 5–2Modulus of Elasticity 5–2Poisson’s Ratio 5–2Modulus of Rigidity 5–3Strength Properties 5–3Common Properties 5–3Less Common Properties 5–15Vibration Properties 5–17Speed of Sound 5–17Internal Friction 5–17Mechanical Properties of Clear Straight-GrainedWood 5–21Natural Characteristics Affecting MechanicalProperties 5–26Specific Gravity 5–26Knots 5–26Slope of Grain 5–28Annual Ring Orientation 5–31Reaction Wood 5–31Juvenile Wood 5–32Compression Failures 5–33Pitch Pockets 5–33Bird Peck 5–33Extractives 5–34Properties of Timber from Dead Trees 5–34Effects of Manufacturing and ServiceEnvironments 5–34Temperature 5–35Time Under Load 5–38Aging 5–41Exposure to Chemicals 5–41Chemical Treatment 5–41Nuclear Radiation 5–43Mold and Stain Fungi 5–43Decay 5–43Insect Damage 5–44Literature Cited 5–44Additional References 5–44The mechanical properties presented in this chapter wereobtained from tests of pieces of wood termed “clear” and“straight grained” because they did not contain characteristics such as knots, cross grain, checks, and splits. These testpieces did have anatomical characteristics such as growthrings that occurred in consistent patterns within each piece.Clear wood specimens are usually considered “homogeneous” in wood mechanics.Many of the mechanical properties of wood tabulated in thischapter were derived from extensive sampling and analysisprocedures. These properties are represented as the average mechanical properties of the species. Some properties,such as tension parallel to the grain, and all properties forsome imported species are based on a more limited numberof specimens that were not subjected to the same samplingand analysis procedures. The appropriateness of these latterproperties to represent the average properties of a speciesis uncertain; nevertheless, the properties represent the bestinformation available.Variability, or variation in properties, is common to allmaterials. Because wood is a natural material and the treeis subject to many constantly changing influences (such asmoisture, soil conditions, and growing space), wood properties vary considerably, even in clear material. This chapterprovides information, where possible, on the nature andmagnitude of variability in properties.This chapter also includes a discussion of the effect ofgrowth features, such as knots and slope of grain, on clearwood properties. The effects of manufacturing and serviceenvironments on mechanical properties are discussed, andtheir effects on clear wood and material containing growthfeatures are compared. Chapter 7 discusses how theseresearch results have been implemented in engineeringstandards.Orthotropic Nature of WoodWood may be described as an orthotropic material; that is,it has unique and independent mechanical properties in thedirections of three mutually perpendicular axes: longitudinal, radial, and tangential. The longitudinal axis L is parallelto the fiber (grain); the radial axis R is normal to the growthrings (perpendicular to the grain in the radial direction); andthe tangential axis T is perpendicular to the grain but tangentto the growth rings. These axes are shown in Figure 5–1.5–1
General Technical Report FPL–GTR–190Table 5–1. Elastic ratios for various species atapproximately 12% moisture contentaFigure 5–1. Three principal axes of wood with respect tograin direction and growth rings.Elastic PropertiesTwelve constants (nine are independent) are needed to describe the elastic behavior of wood: three moduli of elasticity E, three moduli of rigidity G, and six Poisson’s ratios μ.The moduli of elasticity and Poisson’s ratios are related byexpressions of the form(5–1)General relations between stress and strain for a homogeneous orthotropic material can be found in texts onanisotropic elasticity.Modulus of ElasticityElasticity implies that deformations produced by low stressare completely recoverable after loads are removed. Whenloaded to higher stress levels, plastic deformation or failureoccurs. The three moduli of elasticity, which are denotedby EL, ER, and ET, respectively, are the elastic moduli alongthe longitudinal, radial, and tangential axes of wood. Thesemoduli are usually obtained from compression tests; however, data for ER and ET are not extensive. Average valuesof ER and ET for samples from a few species are presented inTable 5–1 as ratios with EL; the Poisson’s ratios are shownin Table 5–2. The elastic ratios, and the elastic constantsthemselves, vary within and between species and with moisture content and specific gravity.The modulus of elasticity determined from bending, EL,rather than from an axial test, may be the only modulus ofelasticity available for a species. Average EL values obtainedfrom bending tests are given in Tables 5–3 to 5–5. Representative coefficients of variation of EL determined withbending tests for clear wood are reported in Table 5–6. Astabulated, EL includes an effect of shear deflection; EL frombending can be increased by 10% to remove this effect approximately. This adjusted bending EL can be used to determine ER and ET based on the ratios in Table 5–1.Poisson’s RatioWhen a member is loaded axially, the deformation perpendicular to the direction of the load is proportional to the5–2SpeciesET/ELAsh, whiteBalsaBasswoodBirch, yellowCherry, blackCottonwood, easternMahogany, AfricanMahogany, HondurasMaple, sugarMaple, redOak, redOak, whiteSweetgumWalnut, blackYellow-poplarBaldcypressCedar, northern whiteCedar, western redDouglas-firFir, subalpineHemlock, westernLarch, edSlashSugarWestern whiteRedwoodSpruce, SitkaSpruce, EngelmannER/ELGLR/ELGLT/EL 80.128aEL may be approximated by increasing modulus of elasticity values inTable 5–3 by 10%.deformation parallel to the direction of the load. The ratioof the transverse to axial strain is called Poisson’s ratio.The Poisson’s ratios are denoted by μLR, μRL, μLT, μTL, μRT,and μTR. The first letter of the subscript refers to directionof applied stress and the second letter to direction of lateraldeformation. For example, μLR is the Poisson’s ratio fordeformation along the radial axis caused by stress alongthe longitudinal axis. Average values of experimentally determined Poisson’s ratios for samples of a few species aregiven in Table 5–2. The ideal relationship between Poisson’sratio and the moduli of elasticity given in Equation (5–1) arenot always closely met. Two of the Poisson’s ratios, μRL andμTL, are very small and are less precisely determined thanare those for other Poisson’s ratios. Poisson’s ratios varywithin and between species and are affected by moisturecontent and specific gravity.
Chapter 5 Mechanical Properties of WoodTable 5–2. Poisson’s ratios for various species atapproximately 12% moisture contentSpeciesµLRAsh, whiteAspen, quakingBalsaBasswoodBirch, yellowCherry, blackCottonwood, easternMahogany, AfricanMahogany, HondurasMaple, sugarMaple, redOak, redOak, whiteSweetgumWalnut, blackYellow-poplarBaldcypressCedar, northern whiteCedar, western redDouglas-firFir, subalpineHemlock, westernLarch, edSlashSugarWestern whiteRedwoodSpruce, SitkaSpruce, EngelmannµLTµTRµRLµTLHardwoods0.371 0.440 0.6840.489 0.374 —0.229 0.488 0.6650.364 0.406 0.9120.426 0.451 0.6970.392 0.428 0.6950.344 0.420 0.8750.297 0.641 0.6040.314 0.533 0.6000.424 0.476 0.7740.434 0.509 0.7620.350 0.448 0.5600.369 0.428 0.6180.325 0.403 0.6820.495 0.632 0.7180.318 0.392 oods0.338 0.326 0.4110.337 0.340 0.4580.378 0.296 0.4840.292 0.449 0.3900.341 0.332 0.4370.485 0.423 0.4420.355 0.276 0.3890.356 ——0.345 ——0.403 ——0.374 0.036 0.0290.336 ——0.382 ——0.352 90.3600.3720.4220.362 ——0.381 ——0.342 ——0.320 ——0.359 ——0.308 ——0.387 ——0.358 ——0.334 ——0.400 ——0.245 0.040 0.0250.255 0.083 .4470.4280.4100.3730.4350.530Modulus of RigidityThe modulus of rigidity, also called shear modulus, indicates the resistance to deflection of a member caused byshear stresses. The three moduli of rigidity denoted by GLR,GLT, and GRT are the elastic constants in the LR, LT, andRT planes, respectively. For example, GLR is the modulusof rigidity based on shear strain in the LR plane and shearstresses in the LT and RT planes. Average values of shearmoduli for samples of a few species expressed as ratios withEL are given in Table 5–1. As with moduli of elasticity, themoduli of rigidity vary within and between species andwith moisture content and specific gravity.Strength PropertiesCommon PropertiesMechanical properties most commonly measured and represented as “strength properties” for design include modulusof rupture in bending, maximum stress in compression parallel to grain, compressive stress perpendicular to grain, andshear strength parallel to grain. Additional measurementsare often made to evaluate work to maximum load in bending, impact bending strength, tensile strength perpendicularto grain, and hardness. These properties, grouped accordingto the broad forest tree categories of hardwood and softwood (not correlated with hardness or softness), are given inTables 5–3 to 5–5 for many of the commercially importantspecies. Average coefficients of variation for these properties from a limited sampling of specimens are reported inTable 5–6.Modulus of rupture—Reflects the maximum load-carryingcapacity of a member in bending and is proportional to maximum moment borne by the specimen. Modulus of ruptureis an accepted criterion of strength, although it is not a truestress because the formula by which it is computed is validonly to the elastic limit.Work to maximum load in bending—Ability to absorbshock with some permanent deformation and more or lessinjury to a specimen. Work to maximum load is a measureof the combined strength and toughness of wood underbending stresses.Compressive strength parallel to grain—Maximum stresssustained by a compression parallel-to-grain specimen having a ratio of length to least dimension of less than 11.Compressive stress perpendicular to grain—Reported asstress at proportional limit. There is no clearly defined ultimate stress for this property.Shear strength parallel to grain—Ability to resist internalslipping of one part upon another along the grain. Valuespresented are average strength in radial and tangential shearplanes.Impact bending—In the impact bending test, a hammer ofgiven weight is dropped upon a beam from successively increased heights until rupture occurs or the beam deflects152 mm (6 in.) or more. The height of the maximum drop,or the drop that causes failure, is a comparative value thatrepresents the ability of wood to absorb shocks that causestresses beyond the proportional limit.Tensile strength perpendicular to grain—Resistance ofwood to forces acting across the grain that tend to split amember. Values presented are the average of radial andtangential observations.Hardness—Generally defined as resistance to indentationusing a modified Janka hardness test, measured by the loadrequired to embed a 11.28-mm (0.444-in.) ball to one-halfits diameter. Values presented are the average of radial andtangential penetrations.Tensile strength parallel to grain—Maximum tensilestress sustained in direction parallel to grain. Relatively fewdata are available on the tensile strength of various species5–3
General Technical Report FPL–GTR–190Table 5–3a. Strength properties of some commercially important woods grown in the United States (metric)aStatic bendingCommon speciesnamesAlder, gBasswood, AmericanBeech, AmericanBirchPaperSweetYellowButternutCherry, blackChestnut, AmericanCottonwoodBalsam 5–4Modulus ModulusofofMoisture Specific rupture elasticityc(kPa)content gravityb(MPa)ComCom- pression Shear TensionWork topression perpen- parallel perpen- SidemaxiImpact parallel diculardicular hardtomumloadbending to grain to grain grain to grain ness(N)(kPa) (kPa)(kPa)(kPa)(kJ m–3) 3,900Errata, 2018: Corrected MOR value for 12% MC white ash.
Chapter 5 Mechanical Properties of WoodTable 5–3a. Strength properties of some commercially important woods grown in the United States (metric)a—con.Static bendingCommon speciesnamesHickory, pecanBitternutNutmegPecanWaterHickory, cust, RedSilverSugarOak, redBlackCherrybarkLaurelNorthern redPinScarletSouthern redWaterMoisture Specificcontent MPa)ComWork toCom- pression Shear Tensionmaxipression perpen- parallel perpen- SidemumImpact parallel dicularto dicular to hardload bending to grain to grain graingrainness(kJ m–3) 08,50011,600—5,2007,10010,800—5,400 10,20011,900 002,3006,8003,9009,2003,2007,2005,900 ,3005–5
General Technical Report FPL–GTR–190Table 5–3a. Strength properties of some commercially important woods grown in the United States (metric)a—con.Static bendingCommon speciesnamesOak, red—con.WillowOak, whiteBurChestnutLiveOvercupPostSwamp chestnutSwamp whiteWhiteSassafrasSweetgumSycamore, AmericanTanoakTupeloBlackWaterWalnut, blackWillow, blackYellow-poplarBaldcypressCedarAtlantic whiteEastern redcedarIncenseNorthern white5–6Moisture Specificcontent MPa)ComWork toCom- pression Shear Tensionmaxipression perpen- parallel perpenmumtoImpact parallel diculardicularload bending to grain to grain grain to grain(kJ m–3) (mm)(kPa) ,9004,0001,7002,1001,0001,40041281035744373933
Chapter 5 Mechanical Properties of WoodTable 5–3a. Strength properties of some commercially important woods grown in the United States (metric)a—con.Static bendingCommon speciesnamesCedar—con.Port-OrfordWestern redcedarYellowDouglas-fireCoastInterior WestInterior NorthInterior SouthFirBalsamCalifornia redGrandNoblePacific arch, westernPineEastern whiteJackLoblollyLodgepoleLongleafPitchMoisture Specificcontent MPa)ComCom- pression Shear TensionWork topression perpen- parallel perpenmaxiImpact parallel diculartodicularmumbending to grain to grain grain to grainload(kPa)(kPa)(kPa) (kPa)(kJ m–3) 1,5002,1002,6003,900——5–7
General Technical Report FPL–GTR–190Table 5–3a. Strength properties of some commercially important woods grown in the United States (metric)a—con.Static bendingCommon afSlashSpruceSugarVirginiaWestern mannRedSitkaWhiteTamarackMoisture Specificcontent MPa)ComCom- pression Shear TensionWork topression perpen- parallel perpenmaxiImpact paralleliculardicularmumtobending to grain to grain grain to grainload(kPa)(kPa)(kJ m–3) (mm)(kPa) aResults of tests on clear specimens in the green and air-dried conditions, converted to metric units directly from Table 5–3b. Definition of properties:impact bending is height of drop that causes complete failure, using 0.71-kg (50-lb) hammer; compression parallel to grain is also called maximumcrushing strength; compression perpendicular to grain is fiber stress at proportional limit; shear is maximum shearing strength; tension is maximum tensilestrength; and side hardness is hardness measured when load is perpendicular to grain.bSpecific gravity is based on weight when ovendry and volume when green or at 12% moisture content.cModulus of elasticity measured from a simply supported, center-loaded beam, on a span depth ratio of 14/1. To correct for shear deflection, the moduluscan be increased by 10%.dValues for side hardness of the true hickories are from Bendtsen and Ethington (1975).eCoast Douglas-fir is defined as Douglas-fir growing in Oregon and Washington State west of the Cascade Mountains summit. Interior West includesCalifornia and all counties in Oregon and Washington east of, but adjacent to, the Cascade summit; Interior North, the remainder of Oregon andWashington plus Idaho, Montana, and Wyoming; and Interior South, Utah, Colorado, Arizona, and New Mexico.5–8
Chapter 5 Mechanical Properties of WoodTable 5–3b. Strength properties of some commercially important woods grown in the United States (inch–pound)aStatic bendingCommon speciesnamesAlder, gBasswood, AmericanBeech, AmericanBirchPaperSweetYellowButternutCherry, blackChestnut, AmericanCottonwoodBalsam, Moisture Specificcontent t parallelofmumelasticitycbending to grainruptureload(lbf in–2) ( 106 lbf in–2) (in-lbf in–3) (in.) (lbf in–2)Compression Shear Tensionperpen- parallel perpen- Sidedicular hardtodicularto grain grain to grain ness(lbf in–2) (lbf in–2) (lbf in–2) 04,7303,5507,3002104
5–2 Elastic Properties Twelve constants (nine are independent) are needed to de-scribe the elastic behavior of wood: three moduli of elastic-ity E