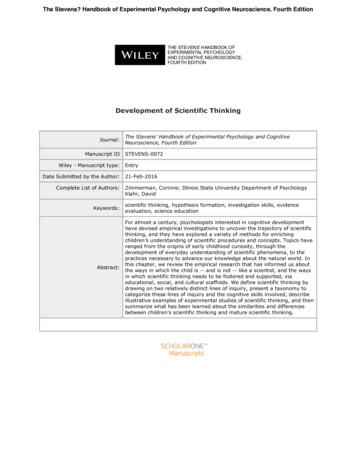
Transcription
The Stevens? Handbook of Experimental Psychology and Cognitive Neuroscience, Fourth EditionDevelopment of Scientific ThinkingJournal:The Stevens’ Handbook of Experimental Psychology and CognitiveNeuroscience, Fourth EditionrFoManuscript IDWiley - Manuscript type:Date Submitted by the Author:Keywords:Entry21-Feb-2016Zimmerman, Corinne; Illinois State University Department of PsychologyKlahr, DavidReComplete List of Authors:STEVENS-0072scientific thinking, hypothesis formation, investigation skills, evidenceevaluation, science educationviewFor almost a century, psychologists interested in cognitive developmenthave devised empirical investigations to uncover the trajectory of scientificthinking, and they have explored a variety of methods for enrichingchildren’s understanding of scientific procedures and concepts. Topics haveranged from the origins of early childhood curiosity, through thedevelopment of everyday understanding of scientific phenomena, to thepractices necessary to advance our knowledge about the natural world. Inthis chapter, we review the empirical research that has informed us aboutthe ways in which the child is -- and is not -- like a scientist, and the waysin which scientific thinking needs to be fostered and supported, viaeducational, social, and cultural scaffolds. We define scientific thinking bydrawing on two relatively distinct lines of inquiry, present a taxonomy tocategorize these lines of inquiry and the cognitive skills involved, describeillustrative examples of experimental studies of scientific thinking, and thensummarize what has been learned about the similarities and differencesbetween children’s scientific thinking and mature scientific thinking.lyOnAbstract:
Page 1 of 48 The Stevens? Handbook of Experimental Psychology and Cognitive Neuroscience, Fourth EditionScientific Thinking1Development of Scientific ThinkingCorinne ZimmermanIllinois State UniversityDavid KlahrCarnegie Mellon UniversityrFoReAuthor NoteviCorinne Zimmerman, Department of Psychology, Illinois State University; David Klahr,ewDepartment of Psychology, Carnegie Mellon University.In preparing this review, we have drawn heavily upon work produced throughcollaborations over the years with several colleagues, including Sharon Carver, Zhe Chen, SteveOnCroker, Anne Fay, Kevin Dunbar, Jamie Jirout, Amy Masnick, Brian Matlen, Bradley Morris,David Penner, Chris Schunn, Martin Schwichow, and Herbert Simon. This chapter could nothave been written without their prior contributions, but we do not hold them responsible for anylyerrors, distortions, or egregious omissions in this chapter.
The Stevens? Handbook of Experimental Psychology and Cognitive Neuroscience, Fourth Edition Page 2 of 48Scientific Thinking2CHAPTER 7: DEVELOPMENT OF SCIENTIFIC THINKINGWhy Study Scientific Thinking?For almost a century, psychologists interested in cognitive development have devisedempirical investigations to uncover the origins and trajectory of scientific thinking and haveexplored a variety of methods for enriching children’s understanding of scientific procedures andconcepts. The study of scientific thinking is particularly appealing to cognitive anddevelopmental psychologists not only because of science’s cultural value, but also because of therFoinherent importance and challenge of investigating scientific thinking and the paradox of “thechild as scientist.” In addition, science educators are interested in the topic because of its obviousCultural ValueviRerelevance for improving science instruction.Science and technology have had profound effects on human culture. Scientific thinkingewhas enhanced the ability of human beings to understand, predict, and control the natural forcesthat shape our world. Scientific literacy refers to the skills required by citizens in a scientificallyOnadvanced society. Students, citizens, and policy makers need to understand how to investigate,evaluate, and comprehend science content (e.g., climate change, evolution, astronomy, disease),lyprocesses (e.g., how to test hypotheses effectively), and products (e.g., from evaluating dataabout the most effective cancer treatments to the possibility of space colonization). In addition tothe “factual” aspects of scientific knowledge and scientific procedures, the well-being of asociety depends on a wide-spread appreciation of the value of science (e.g., the necessity ofevidence-based decisions about policies, practices, and programs). A report from the NationalResearch Council (NRC; 2010) argues that science is the discipline that should be used to
Page 3 of 48 The Stevens? Handbook of Experimental Psychology and Cognitive Neuroscience, Fourth EditionScientific Thinking3convey the skills required for the 21st century workforce, such as non-routine problem solving,adaptability, complex communication skills, self-management, and systems thinking.Inherent Importance and DifficultyAlthough scientific thinking has its roots in “everyday thinking,” it is much morecomplex, highly structured, and refined. There has been a rich mythology about the importance,on one hand, and the intractability, on the other, of studying the psychological processes that leadto scientific discovery. With respect to intractability, Einstein once mused, “I am not surerFowhether there can be a way of really understanding the miracle of thinking” (Wertheimer, 1959p. 227). If Einstein’s bewilderment were correct then this chapter could conclude right here.ReHowever, in a more optimistic and constructive reflection, Einstein also said:The whole of science is nothing more than a refinement of everyday thinking. It is forvithis reason that the critical thinking of the physicist cannot possibly be restricted to theexamination of concepts of his own specific field. He cannot proceed withoutewconsidering critically a much more difficult problem, the problem of analyzing thenature of everyday thinking.” (Einstein, 1936, p.59)OnIn the decades since Einstein had this remarkable insight, cognitive scientists have madesubstantial advances in understanding the “nature of everyday thinking.” At the same time, therelyhas been a rich and active line of research on the cognitive and social processes involved in inscientific inquiry and discovery. Thus, the very difficulty of studying this complex, multilayered,and socially impactful topic provides a strong justification for the endeavor.The Paradox of the “Child as Scientist”Both children and scientists are described as “naturally curious” with an inherent andenthusiastic approach to finding out about the world. Research shows that infants and youngchildren have some of the precursors and abilities needed to engage in formal scientific thinking.At the same time, older children and adults struggle with scientific thinking tasks; they can be
The Stevens? Handbook of Experimental Psychology and Cognitive Neuroscience, Fourth Edition Page 4 of 48Scientific Thinking4unsystematic, ignore and misinterpret evidence, try to prove what they already believe to be true,and design uninformative experiments (just to name a few of the documented difficulties).Although U- and inverted-U-shaped curves are common in developmental psychology, there ismore to this story than early and late competence. By systematically studying age-appropriatetasks across the lifespan, we have learned a lot about the extent to which the child-as-scientistview is supported, and the extent to which, from pre-school to college, people have deep andwell-entrenched misconceptions about both the content and processes of science.rFoEducational RelevanceThe study of basic cognitive processes involved in scientific thinking has obvious andReimportant implications for science education across the curriculum, from pre-Kindergartenthrough college. For example, Piaget’s theory of cognitive development was very influential invithe design and development of science curricula from the late 1950’s through the end of the lastewcentury (Blake & Pope, 2008; Elkind, 1972; Kamii, & DeVries, 1993; Klahr, 2012; Metz, 1995,1997). And even as the field of cognitive development has distanced itself from much of Piaget’sOntheoretical edifice, many current educational practitioners, from K through college level,continue to base much of their instruction upon Piagetian stages of cognitive development.lyScience education (in the US in particular) has undergone remarkable change within the pastseveral decades (DeBoer, 2000). Recently, in the US, national organizations such as the NationalResearch Council have been focused on evidence-based efforts to improve science education.These efforts have included an explicit acknowledgement of the basic psychological research onscientific thinking (e.g., NRC, 2000, 2007, 2008). The “Taking Science to School K-8” report(NRC, 2007) has emphasized and summarized several important emergent themes in recentresearch in the learning sciences and cognitive development. For example, with respect to
Page 5 of 48 The Stevens? Handbook of Experimental Psychology and Cognitive Neuroscience, Fourth EditionScientific Thinking5summarizing what research has told us about how science is learned, the “surprisinglysophisticated” nature of children’s thinking is acknowledged, as is their ability to engage in avariety of reasoning processes that represent the precursors to mature scientific thinking. Therole of adults, teachers, and learning experiences is emphasized as a way to turn children’s “richbut naïve understandings of the natural world” (NRC, 2007, p. 3) into proficient skills needed forengaging in investigation, evidence evaluation, explanation, argumentation, and discourse asscience students and as scientifically literate adults. Recommendations about best practices forrFosupporting this transition have been proposed (NRC, 2007, 2008), which acknowledge children’sexisting and developing capabilities, focus on core knowledge areas and skills, and promote theReopportunity for the students to engage in the practices of science.The plan for this chapter is as follows. We will (1) define scientific thinking by drawingvion two relatively distinct lines of inquiry; (2) present a taxonomy to categorize these lines ofewinquiry and the cognitive skills involved; (3) describe illustrative examples of experimentalstudies of scientific thinking; and finally (4) summarize what has been learned about theOnsimilarities and differences between children’s scientific thinking and mature scientific thinking.What is Scientific Thinking?lyScience, as a human endeavor, can be approached as an individual, social, and culturalactivity. At the individual level, scientific thinking shares many characteristics with other formsof problem solving and reasoning (Klahr, Matlen & Jirout, 2013; Zimmerman & Croker, 2013).It can be further described as a specific type of intentional information or knowledge seeking(Kuhn, 2011). Curiosity emerges early and spontaneously in children (Jirout & Klahr, 2012).However, before this innate curiosity can effectively address scientific issues, it must be refinedand shaped by instruction through deliberate activities such as exploring, asking questions,
The Stevens? Handbook of Experimental Psychology and Cognitive Neuroscience, Fourth Edition Page 6 of 48Scientific Thinking6testing hypotheses, engaging in inquiry, and evaluating evidence (Jirout & Zimmerman, 2015;Morris, Croker, Masnick, & Zimmerman, 2012). An additional defining feature of maturescientific thinking involves metacognitive and metastrategic knowledge – the ability to reflect onthe process of knowledge acquisition and the changes that result from engaging in scientificactivities (Kuhn, 2011). Metacognitive skills are evident in children’s emerging theory-of-mindskills. Children must learn where beliefs about the world around them come from, that othersmay have different beliefs, that beliefs can be more or less certain, and in particular – that beliefsrFomay be formed on the basis of inference or from evidence (e.g., Sodian & Wimmer, 1987). Thisbroad and encompassing definition provides a context in which to summarize a very wide rangeReof investigations, from studies of young children making observations in a school classroom(e.g., Chinn & Malhotra, 2002) to descriptions of a research team in a laboratory discussing theviresults of a set of experiments (e.g., Dunbar, 1995).ewPsychological studies of scientific thinking have taken several forms, including historicalaccounts and case studies of individual scientists or groups of scientists (see Klahr, 1994; KlahrOn& Simon, 1999 for review) and computational models of the cognitive processes underlyingscientific problem solving and discovery (see Shrager & Langley, 1990 for overview). In thislychapter, we focus on psychological studies of participants in simulated discovery contexts.Participants from some characteristic population (e.g., school children, college students,scientists) are presented with problem-solving situations that isolate one or more essentialaspects of “real world” science. The “thing-to-be-discovered” can range from something assimple and arbitrary as a “rule” that the experimenter has in mind (e.g., Wason, 1960) tosomething as complex as the physics of an artificial universe (Mynatt, Doherty & Tweney, 1977)or the mechanisms of genetic inhibition (Dunbar, 1993). The advantage of this approach is that it
Page 7 of 48 The Stevens? Handbook of Experimental Psychology and Cognitive Neuroscience, Fourth EditionScientific Thinking7enables the researcher to exert some experimental control over participants’ prior knowledge andcomplete control over the “state of nature.” Most important, this approach enables the researcherto observe the dynamic course of scientific discovery processes in great detail.Conceptual Thinking in ScienceThe beginning of psychological research on scientific thinking has been widely attributedto Jean Piaget’s meeting with Albert Einstein in 1928. Einstein was curious about thedevelopmental origins of fundamental physical concepts. Piaget began a line of research thatrFoincluded children’s understanding of scientific concepts and their skills for investigating theworld and credits Einstein for inspiration (Piaget 1946/1969). Piaget investigated children’sRedeveloping thinking processes about time, speed, distance, number, movement, velocity, livingthings, people, space, mathematics, logic, morality, physical causality, and psychology. Thevilegacy of his steadfast focus on children’s early understanding of causes and effects in theewnatural world cannot be overstated. Piaget’s influence is eloquently summarized by John Flavell(1996), and continues to be reiterated (Klahr, 2012).OnFollowing on in this tradition of studying scientific thinking, researchers have examinedthe concepts that children and adults hold in the various domains of science, such as biology (e.g.,lyCarey, 1985; Hatano & Inagaki, 2013), chemistry (Calik & Ayas, 2005; Garnett, Garnett, &Hackling, 1995), and physics (e.g., Chi, Feltovich, & Glaser, 1981).Numerous examples ofspecific concepts within these domains have been studied. For example, conceptual developmenthas been studied in astronomy (Vosnaiadou & Brewer, 1994), gravity (Hood, 1998), genetics(Echevarria, 2003), evolution (Emmons & Kelemen, 2015; Samarapungavan & Wiers, 1997),ecology (Zimmerman & Cuddington, 2007), earth and space concepts (Sackes, 2015), life
The Stevens? Handbook of Experimental Psychology and Cognitive Neuroscience, Fourth Edition Page 8 of 48Scientific Thinking8science concepts (Akerson, Wieland, & Fouad, 2015), buoyancy, current, and bubbles(Tenenbaum, Rappolt-Schlichtmann, & Zanger, 2004).The focus in this line of research has been to identify and describe the mental models ordomain-specific theories that children and adults hold about scientific phenomena and theprogression of changes that these models undergo with experience or instruction. Here, scientificthinking is studied by asking participants to use their conceptual knowledge about particularscientific phenomena to answer questions and reason about novel scenarios. Two classic studiesrFoillustrate this type of scientific thinking. To probe children’s understanding of the shape of theearth, Vosniaduou and Brewer (1992) asked 6- to 11-year-olds factual questions such as “WhatReis the shape of the earth?” and questions that would differentiate children’s conceptualizations,such as “What is above/below the earth?” and “Can you fall off the edge [of the earth]?” Theseviresponses, along with drawings, uncovered a variety of alternative mental models that varied inewinternal consistency (e.g., disc earth, hollow sphere, rectangular earth). In the domain of genetics,Clough and Driver (1986) asked early adolescents to reason about situations of geneticOninheritance involving the offspring of humans or animals with acquired traits (e.g., a gardenerwith rough skin, a mouse that had lost its tail). Developmental trends in beliefs about inheritancelyand accompanying explanations were evident from 12 to 16 years of age.The vast number of possible concepts that can be investigated makes it difficult toadequately summarize the findings from this literature, because of the domain-specific nature ofsuch research. For example, as of 2009, Reinders Duit, a researcher at University of Kiel,compiled a bibliography of research studies on conceptual change in science with more than8,400 entries.1 Consider, for example, a single chapter on children’s understanding of physicalscience concepts. Hadzigeorgiou (2015) reviews studies about children’s ideas about matter, heat,1For last update see http://archiv.ipn.uni-kiel.de/stcse/.
Page 9 of 48 The Stevens? Handbook of Experimental Psychology and Cognitive Neuroscience, Fourth EditionScientific Thinking9temperature, evaporation, condensation, the water cycle, forces, motion, floating, sinking,electricity, and light. Each of these topics can be further unpacked to constituent subcomponents(e.g., electricity concepts include current, voltage, charge, electrons, resistance, and circuits, toname a few).In the most current science standards in the USA, the NRC (2012) has identified threedimensions that represent “a broad set of expectations for students in science” (p. 1), includingDisciplinary Core Ideas, Crosscutting Concepts, and Scientific and Engineering Practices. TherFocore ideas are restricted to the traditional sciences (i.e., physical, life, earth/space). Thecrosscutting concepts, in contrast, are broadly applicable to several domains of science, andReinclude ideas such as causality, patterns, time, feedback, analogy, and equilibrium. There is arich literature on what individuals understand about the concept of causality across the lifespan,vifrom infancy studies (e.g., Baillargeon, 2004) through childhood (e.g., Gopnik, Sobel, Schulz &ewGlymour, 2001; Piaget, 1974) and adulthood (for a review see Koslowski & Masnick, 2010).Although less work has been done on the other domain-general crosscutting concepts, there are aOnfew exceptions. Swanson (2015) examined students’ understanding of patterns (as a process orbehavior) that can underlie physical, social, or psychological phenomena (e.g., threshold,Procedural Thinking in Sciencelyequilibration, and oscillation) in a middle-school science course called “the Patterns Class.”In addition to studies addressing what children know about science, there is a substantialliterature on how children acquire that knowledge. Here too, Piaget led the way in his decision tostudy not only what children know about the world at various stages of their development, butalso, and perhaps more importantly, the methods and processes that they use to acquire,integrate, and refine this knowledge. This second line of research examines the development of
The Stevens? Handbook of Experimental Psychology and Cognitive Neuroscience, Fourth Edition Page 10 of 48Scientific Thinking 10scientific thinking by focusing on the scientific practices of observing, asking questions,conducting experiments, evaluating evidence, constructing models, and generating explanations.In this line of investigation, “scientific thinking is something people do, not something they have”(Kuhn, 2011, p. 498).The distinction between the products and processes of scientific thinking is reflected inthe often distinct research programs that developmental psychologists have undertaken. It ispossible to develop a line of research about children’s knowledge about various content (e.g.,rFoastronomy, biology) without needing to be concerned about their investigation skills (i.e., howthey came to know it). For example, Vosiadou and Brewer’s (1992) study of children’sReconceptions about the shape of the earth is a canonical study of scientific knowledge, but it doesnot address children’s knowledge-producing activities. Studies of such knowledge-producingviinvestigation skills have utilized two main strategies. One is to reduce the reliance on (orewinterference by) conceptual knowledge by creating tasks that reduce the role of prior knowledge(e.g., Wason, 1960; Siegler & Liebert, 1975). The other is to examine these knowledge types in aauthentic scientific thinking.Onmore integrated way, motivated by the fact that concepts and procedures are intertwined inlyA Taxonomy for Categorizing Experimental Studies of Scientific ThinkingOur taxonomy utilizes the two principle features that have been used previously tocharacterize scientific thinking: domain-specific concepts and domain-general procedures(Klahr, 1994; Klahr, Zimmerman, & Jirout, 2011; Zimmerman, 2000; 2007). In addition, Table 1extends this taxonomy to incorporate some of the Scientific and Engineering Practices that havebeen identified by the NRC’s (2012) national science education standards. The cognitiveprocesses identified by Klahr and Dunbar (1988) are included in the first column. The scientific
Page 11 of 48 The Stevens? Handbook of Experimental Psychology and Cognitive Neuroscience, Fourth EditionScientific Thinking 11practices that map on to these cognitive processes are included in the second column. In the thirdand fourth columns, we distinguish between domain-general and domain-specific knowledgebecause some studies focus on specific content whereas others use simple or abstract contextsthat do not require much (or any!) domain-specific knowledge. For example, a typical domainspecific study might investigate what children understand about the domain of chemistry byasking them to reason about processes such as dissolution and chemical change (Calik & Ayas,2005). Domain-general studies, in contrast, focus on broadly applicable reasoning processes thatrFocan be investigated in arbitrary and abstract forms, such as Bruner’s classic concept learningtasks (Bruner, Goodnow, & Austin, 1956) or Wason’s famous 2-4-6 task (Wason, 1960).ReSome preliminaries are in order. Some studies might fit neatly into a single cell, but forthe majority of this literature (described in more detail in the next section), even studies thatvifocus on a particular cognitive process or scientific practice have to traverse more than one cell.ewFor example, in order to examine experimentation skills, participants must set up (or, select froma pre-defined set of choices) an experiment to address a particular hypothesis. Likewise, theOnevaluation of evidence must be done in some context, and requires a consideration of either theexperiment that produced it, or the hypothesis that it is meant to support or refute.lyEmpirical Investigations of the Development of Scientific ThinkingKlahr and Dunbar’s (1988) Scientific Discovery as Dual Search (SDDS) model will serveas the general framework for organizing illustrative empirical findings to be discussed (see Table1). The SDDS framework captures the complexity and the cyclical nature of the process ofscientific discovery and includes both inquiry skills and conceptual change (see Klahr, 2000, fora detailed discussion). The top-level categories of the model include the three major cognitivecomponents of scientific discovery: Searching for hypotheses, searching for experiments (or
The Stevens? Handbook of Experimental Psychology and Cognitive Neuroscience, Fourth Edition Page 12 of 48Scientific Thinking 12investigations more generally), and evidence evaluation. The studies to be reviewed involve oneor more of these three processes. SDDS is an extension of a classic model of problem solvingfrom the field of cognitive science (Newell & Simon, 1972, Simon & Lea, 1974) and explainshow people carry out problem solving in varied science contexts, from simulated inquiry toprofessional scientific practice. The fundamental aspects of SDDS are (a) the concept of twodistinct, but closely related, “problem spaces”: a space of hypotheses and a space of experiments;and (b) coordinated search in these two problem spaces.rFoIndividuals begin inquiry tasks with some existing or intuitive ideas, or perhaps no ideasat all about how particular variables influence an outcome. Given some set of possible variablesRe(i.e., independent variables) and asked to determine their effect on an outcome (i.e., thedependent variable), participants negotiate the process by coordinating search in the set ofvipossible hypotheses and the set of possible experiments. Experiments are conducted to determineewthe truth status of the current hypothesis, or to decide among a set of competing hypotheses.Experiments may also be conducted to generate enough data to be able to propose a hypothesisOn(as might be the case when one has little or no prior knowledge). Evidence is then evaluated sothat inferences can be made whether a hypothesis is correct or incorrect (or, in some cases, thatlythe evidence is inconclusive). Depending on the complexity of the task, the number of variables,and the amount of time on task, these processes may be repeated several times as an individualnegotiates search in the hypothesis and experiment spaces and makes inferences based on theevaluation of self-generated evidence. Factors such as task domain, amount of prior knowledge,and the perceived goal of the task influence how these cognitive processes are deployed.
Page 13 of 48 The Stevens? Handbook of Experimental Psychology and Cognitive Neuroscience, Fourth EditionScientific Thinking 13Searching the Hypothesis SpaceOf the three cognitive processes of SDDS, search in the hypothesis space has the most incommon with conceptual thinking in science, as it typically involves a search of relevantdomain-specific knowledge as represented in the hypothesis space. When one is engaged ininquiry or investigation activities, hypothesis-space search is instantiated in the service of thescientific practices of asking questions and developing or using models (NRC, 2012).Asking questions and curiosity. Asking questions is one of the foundational processrFoskills of scientific practice (NRC, 2012). Older students often believe that the goal of science isto demonstrate what is already known (Kuhn, 2005), or to see if something “works” or to inventRethings (Carey, Evans, Honda, Jay, & Unger, 1989). However, asking questions for which theanswer is not yet known is a crucial element of inquiry that students must learn (Kuhn & Dean,vi2005). They must learn not only how to ask “good” questions, but also that question-asking is aewdefining feature of science. An essential precursor to asking good questions is curiosity (Jirout &Klahr, 2012; Klahr, Zimmerman, & Jirout, 2011). Curiosity’s fundamental importance in scienceOneducation is indicated by its nearly universal inclusion across a variety of highly influentialscience curricula, educational standards, and assessment goals (AAAS, 1993; National EducationlyGoals Panel, 1995; NAEYC, 2011; NRC, 2000). Curiosity is the desire or motivation to exploreand ask questions. Specifically, we define curiosity as the preferred level of uncertainty – or theamount of uncertainty that will lead to deliberate question asking or exploratory behavior (Jirout& Klahr, 2012, 2016; Jirout & Zimmerman, 2015).Simple problem-solving tasks that require question asking have been used forinvestigating children’s ability to recognize specific instances of uncertainty and to evaluateinformation. Referential tasks assess children’s general ability to ask categorical questions (“is it
The Stevens? Handbook of Experimental Psychology and Cognitive Neuroscience, Fourth Edition Page 14 of 48Scientific Thinking 14an animal?”, “does it bark?”) that will help them to identify a target from a group of possibilities(e.g., one picture from an array of pictures). When children are given the opportunity to ask aquestion to figure out which of the objects is hidden in a box before guessing, they are correct onabout five of the six trials; if they are told to guess what’s in the box without being allowed toask a question, their accuracy is at chance (Chouinard, 2007). Thus, children can determinewhich questions to ask to address uncertainty, but they can also use information yielded by theanswer to their questions to resolve it.rFoResearch on conceptual thinking in science has used various methodologies (drawings,standardized interviews, reasoning scenarios) to uncover children’s understanding of variousRephenomena. At this intersection with process skills, there are examples of children’s questionasking in particular domains. For example, Greif, Kemler Nelson, Keil, and Gutierrez (2006)viinvestigated young children’s ability to ask domain-specific questions on a structured task.ewChildren were instructed to ask questions about unfamiliar objects and animals, which they wereable to do – averaging 26 questions asked across 12 pictures. Many questions were quite general,Onsuch as “what is it?” Other questions, however, showed that children recognized and understoodthat different questions
The Stevens’ Handbook of Experimental Psychology and Cognitive Neuroscience, Fourth Edition Manuscript ID STEVENS-0072 Wiley - Manuscript type: Entry Date Submitted by the Author: 21-Feb-2016 Complete List of Authors: Zimmerman, Corinne; Illinois State University Departm