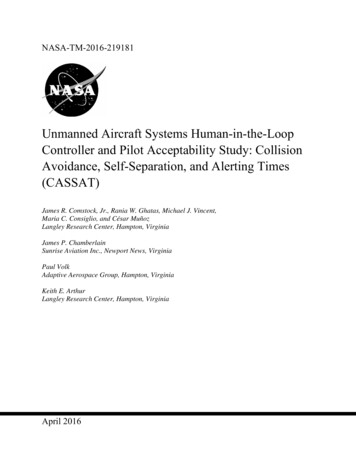
Transcription
NASA-TM-2016-219181Unmanned Aircraft Systems Human-in-the-LoopController and Pilot Acceptability Study: CollisionAvoidance, Self-Separation, and Alerting Times(CASSAT)James R. Comstock, Jr., Rania W. Ghatas, Michael J. Vincent,Maria C. Consiglio, and César MuñozLangley Research Center, Hampton, VirginiaJames P. ChamberlainSunrise Aviation Inc., Newport News, VirginiaPaul VolkAdaptive Aerospace Group, Hampton, VirginiaKeith E. ArthurLangley Research Center, Hampton, VirginiaApril 2016
NASA STI Program . . . in ProfileSince its founding, NASA has been dedicated to theadvancement of aeronautics and space science. TheNASA scientific and technical information (STI)program plays a key part in helping NASA maintainthis important role. CONFERENCE PUBLICATION.Collected papers from scientific and technicalconferences, symposia, seminars, or othermeetings sponsored orco-sponsored by NASA.The NASA STI program operates under the auspicesof the Agency Chief Information Officer. It collects,organizes, provides for archiving, and disseminatesNASA’s STI. The NASA STI program provides accessto the NTRS Registered and its public interface, theNASA Technical Reports Server, thus providing oneof the largest collections of aeronautical and spacescience STI in the world. Results are published in bothnon-NASA channels and by NASA in the NASA STIReport Series, which includes the following reporttypes: SPECIAL PUBLICATION. Scientific,technical, or historical information from NASAprograms, projects, and missions, oftenconcerned with subjects having substantialpublic interest. TECHNICAL TRANSLATION.English-language translations of foreignscientific and technical material pertinent toNASA’s mission. TECHNICAL PUBLICATION. Reports ofcompleted research or a major significant phase ofresearch that present the results of NASAPrograms and include extensive data or theoreticalanalysis. Includes compilations of significantscientific and technical data and informationdeemed to be of continuing reference value.NASA counter-part of peer-reviewed formalprofessional papers but has less stringentlimitations on manuscript length and extent ofgraphic presentations.TECHNICAL MEMORANDUM.Scientific and technical findings that arepreliminary or of specialized interest,e.g., quick release reports, workingpapers, and bibliographies that contain minimalannotation. Does not contain extensive analysis.CONTRACTOR REPORT. Scientific andtechnical findings by NASA-sponsoredcontractors and grantees.Specialized services also include organizingand publishing research results, distributingspecialized research announcements and feeds,providing information desk and personal searchsupport, and enabling data exchange services.For more information about the NASA STI program,see the following: Access the NASA STI program home page athttp://www.sti.nasa.gov E-mail your question to help@sti.nasa.gov Phone the NASA STI Information Desk at757-864-9658 Write to:NASA STI Information DeskMail Stop 148NASA Langley Research CenterHampton, VA 23681-2199
NASA-TM-2016-219181Unmanned Aircraft Systems Human-in-the-LoopController and Pilot Acceptability Study: CollisionAvoidance, Self-Separation, and Alerting Times(CASSAT)James R. Comstock, Jr., Rania W. Ghatas, Michael J. Vincent,Maria C. Consiglio, and César MuñozLangley Research Center, Hampton, VirginiaJames P. ChamberlainSunrise Aviation Inc., Newport News, VirginiaPaul VolkAdaptive Aerospace Group, Hampton, VirginiaKeith E. ArthurLangley Research Center, Hampton, VirginiaNational Aeronautics andSpace AdministrationLangley Research CenterHampton, Virginia 23681-2199April 2016
The use of trademarks or names of manufacturers in this report is for accurate reporting and does notconstitute an official endorsement, either expressed or implied, of such products or manufacturers by theNational Aeronautics and Space Administration.Available from:NASA STI Program / Mail Stop 148NASA Langley Research CenterHampton, VA 23681-2199Fax: 757-864-6500
Table of ContentsTable of Tables . ivTable of Figures . ivList of Acronyms . vAbstract . 1Introduction . 1Phase I – Air Traffic Controller Acceptability Study . 31.Phase I General Information . 31.12.Approach and Objectives . 3Phase I Method . 32.1Subjects . 32.2Procedure . 42.3Independent Variables . 42.4Scenarios . 52.5Communications, Navigation, and Surveillance Assumptions . 62.6Software, Hardware, and Facilities . 72.7Dependent Variables . 72.7.1 Horizontal Miss Distance. . 72.7.2 Alerting Times. . 82.7.3 Workload Assessment. . 82.7.4 System Performance Metrics. . 92.7.5 Post-run Questionnaires. . 93.Phase I Results . 93.1 Horizontal Miss Distance Ratings . 93.2 Alerting Times Ratings . 114.Phase I Discussion . 13Phase II – Pilot Acceptability Study . 145.Phase II General Information . 145.16.Approach and Objectives . 14Phase II Method . 146.1Subjects . 146.2Procedure . 156.3Independent Variables . 15i
6.4Scenarios . 176.5Communications, Navigation, and Surveillance Assumptions . 176.6Software, Hardware, and Facilities . 176.7Dependent Variables . 176.7.1 Horizontal Miss Distance (DTHR). . 176.7.2 Alert Times Ratings. . 176.7.3 Workload Assessment. . 176.7.4 System Performance Metrics. . 176.7.5 Secondary Task. . 186.7.6 Post-encounter Questionnaires. 186.7.7 Post-run Questionnaires. . 187.Phase II Results . 187.1Horizontal Miss Distance Ratings . 187.2Alert Times Ratings . 207.3Distance from Well Clear Boundary . 227.4Vertical Encounters . 267.5Alerting Structures . 288.Phase II Discussion . 289.Conclusion . 29Acknowledgements . 30References . 31Appendix A – Air Traffic Operations Laboratory (ATOL) CASSAT Layout . 32Appendix B – Phase I Initial Questionnaire . 33Appendix C – Encounter Geometries from Phase I and Phase II . 34Head-on Encounter Geometry . 34Crossing Encounter Geometry . 35Overtake Encounter Geometry . 36Vertical-Crossing Encounter Geometry . 36Vertical-Overtake Encounter Geometry . 37Appendix D – Phase I Workload Ratings by Test Hour . 38Appendix E – Phase I End of Hour Questionnaire . 39Appendix F – Phase II Initial Questionnaire. 41Appendix G – Phase II Secondary Task Questions for Sessions 1-3 . 43Appendix H – Phase II Post-encounter Questionnaire . 49ii
Appendix I – Phase II Post-run Questionnaire . 50iii
Table of TablesTable 1 - Independent Variables for Phase I . 5Table 2 - Rating scale definitions used for assessment of Horizontal Miss Distance (HMD) . 8Table 3 - Rating scale definitions used for assessment of Maneuver Request Timing. . 8Table 4 - Independent Variables for Phase II . 16Table 5 - Cases where CPA was less than HMD . 25Table of FiguresFigure 1. Chart showing McKinney National (KTKI), upper right; DFW is in the lower left. . 6Figure 2. Controller ratings for Crossing Geometry Encounters by Horizontal Miss Distance. . 10Figure 3. Controller ratings for Overtake Geometry Encounters by Horizontal Miss Distance. . 10Figure 4. Controller ratings for Head-on Geometry Encounters by Horizontal Miss Distance. 11Figure 5. Controller miss distance ratings by UAS alert times of 30, 45, and 75 seconds. . 12Figure 6. Controller timing rating by UAS alert times of 30, 45, and 75 seconds. . 12Figure 7. Alerting Structure "A” .15Figure 8. Alerting Structure "B” .16Figure 9. UAS Pilot ratings for Crossing Geometry Encounters . 19Figure 10. UAS Pilot ratings for Overtake Geometry Encounters . 19Figure 11. UAS Pilot ratings for Head-on Geometry Encounters. . 20Figure 12. UAS Pilot ratings for Crossing Geometry Encounters . 21Figure 13. UAS Pilot ratings for Overtake Geometry Encounters . 21Figure 14. UAS Pilot ratings for Head-on Geometry Encounters . 22Figure 15. Mean distance from Horizontal Miss Distance at CPA for Crossing GeometryEncounters. 23Figure 16. Mean distance from Horizontal Miss Distance at CPA for Overtake GeometryEncounters. 23Figure 17. Mean distance from Horizontal Miss Distance at CPA for Head-on GeometryEncounters. 24Figure 18. UAS Pilot Distance ratings for Vertical Geometry Encounters for each Alert Time.All HMDs were 0.7 for Vertical Geometry Encounters . 26Figure 19. UAS Pilot Alert Time ratings for Vertical Geometry Encounters . 27Figure 20. Mean distance from Horizontal Miss Distance at CPA for Vertical GeometryEncounters. 27iv
List of SSSSVTCASTCOATRACONUAUASVFRVHFAlerting TimesAir Traffic ControlAir Traffic Operations LaboratoryAir Traffic Workload Input TechniqueCollision AvoidanceController Acceptability StudyCollision Avoidance, Self-Separation, and Alerting TimesCode of Federal RegulationsCommunication, Navigation, and SurveillanceClosest Point of ApproachDetect and AvoidDetect and Avoid Alerting Logic for Unmanned SystemsDallas-Fort WorthDistance-thresholdFederal Aviation AdministrationGround Control StationHorizontal Miss DistanceInstrument Flight RulesAirport Identifier for Collin County Airport (aka McKinney National Airport)Multi Aircraft Control SystemMillisecondNational Airspace SystemNational Aeronautics and Space AdministrationNautical MileResolution Advisory (from TCAS)Radio Technical Commission for AeronauticsSelf-SeparationSelf-Separation VolumeTraffic Alert and Collision Avoidance SystemTime to Co-AltitudeTerminal Radar Approach Control FacilityUnmanned AircraftUnmanned Aircraft SystemsVisual Flight RulesVery High Frequencyv
AbstractThe Federal Aviation Administration (FAA) has been mandated by theCongressional funding bill of 2012 to open the National Airspace System(NAS) to Unmanned Aircraft Systems (UAS). With the growing use ofunmanned systems, NASA has established a multi-center “UASIntegration in the NAS” Project, in collaboration with the FAA andindustry, and is guiding its research efforts to look at and examine crucialsafety concerns regarding the integration of UAS into the NAS. Keyresearch efforts are addressing requirements for detect-and-avoid (DAA),self-separation (SS), and collision avoidance (CA) technologies. In one ofa series of human-in-the-loop experiments, NASA Langley ResearchCenter set up a study known as Collision Avoidance, Self-Separation, andAlerting Times (CASSAT). The first phase assessed active air trafficcontroller interactions with DAA systems and the second phase examinedreactions to the DAA system and displays by UAS Pilots at a simulatedground control station (GCS). Analyses of the test results from Phase Iand Phase II are presented in this paper. Results from the CASSAT studyand previous human-in-the-loop experiments will play a crucial role in theFAA’s establishment of rules, regulations, and procedures to safely,efficiently, and effectively integrate UAS into the NAS.IntroductionUnmanned Aircraft Systems (UAS) have become the forefront of aviation technology and willsoon be commonplace in the National Airspace System (NAS) as a result of the Congressionalfunding bill of 2012, which mandated the Federal Aviation Administration (FAA) to open the NASto UAS. In response to this effort, and with safety being the primary concern, the NationalAeronautics and Space Administration (NASA) has established a “UAS Integration in the NAS”project that spans four NASA centers, in collaboration with the FAA and industry, to examineessential safety concerns regarding the integration of UAS in the NAS. Routine access to the NASwill require UAS to have new equipage, minimum operations standards, rules and regulations, andprocedures. Many supporting research efforts will be required to answer difficult questionsconcerning these standards, regulations, and procedures. Detect-and-avoid (DAA)implementations, self-separation (SS) procedures, and collision avoidance (CA) technologies toremain well-clear of other aircraft are top research priorities in assuring safe integration. Researchefforts at NASA Langley Research Center are guiding the answer to those difficult questions toassure safe and efficient integration of UAS into the NAS. The present study focuses on DAAsystem acceptability to both air traffic controllers and pilots of UAS and is entitled CollisionAvoidance, Self-Separation, and Alerting Times (CASSAT) human-in-the-loop experiment – atwo-phase study and the third in a series of Controller Acceptability Studies (CAS).Many hurdles accompany the safe integration of UAS in the NAS, including the requirement tosee-and-avoid other aircraft per Title 14 of the United States Code of Federal Regulations (CFR)14, Parts 91.111 and 91.113 and other applicable regulations and accepted practices. Pilots arerequired to follow right-of-way rules and remain well clear of other aircraft. In all airspace classes,pilots are expected to comply with these see-and-avoid requirements while also complying withAir Traffic Control (ATC) instructions and clearances or to negotiate changes to these instructions1
and/or clearances as necessary. See-and-avoid capable pilots are generally expected to maneuverand communicate in predictable ways and in a manner that preserves the safety, orderliness, andefficiency of the Airspace system when operating in a positive control environment. UAS willlikely be expected to operate in a similar manner, but with DAA replacing the see-and-avoidcapability of a manned aircraft. The acceptable design space and capabilities for DAA systems inthis environment are largely undefined. CAS-1 and CAS-2 controller-in-the-loop simulationexperiments sought to illuminate the DAA design space for UAS operating in a positive controlATC environment. Reports on the CAS-1 study may be found in Chamberlain, Consiglio,Comstock, Ghatas, and Muñoz (2015), and Ghatas, Comstock, Consiglio, Chamberlain, andHoffler (2015). Reports on the CAS-2 study may be found in both a NASA TechnicalMemorandum (TM), Comstock, Ghatas, Consiglio, Chamberlain, and Hoffler (2015), and in aconference proceedings paper, Comstock, Ghatas, Consiglio, Chamberlain, and Hoffler (2015).As an extension of CAS-1 and CAS-2, CASSAT focused on addressing minimum and maximumacceptable declaration times for projected well clear losses from the perspective of active air trafficcontrollers and of Instrument Flight Rules (IFR) rated pilots and explored alerting structures asDAA and CA functions are integrated.The DAA technology employed in the present study worked much like the algorithms in the TrafficAlert and Collision Avoidance System (TCAS), but with a Self-Separation Volume (SSV), alsoreferred to as the well-clear volume, that was large enough to avoid (a) corrective ResolutionAdvisories (RAs) for TCAS equipped intruders; (b) safety concerns for controllers; and, (c) undueconcern for proximate see-and-avoid pilots. The present series of studies sought to determineoperationally acceptable SSV sizes and look-ahead prediction times to inform system designersabout required DAA surveillance range and accuracy. Guidance from the DAA system wasprovided to the UAS pilot to maintain positions outside the well-clear boundary. Details of theself-separation guidance shown to the UAS pilots to maintain well-clear may be found in the CAS1 paper (Chamberlain, et al., 2015).In addition to avoiding the issuance of TCAS RAs, the DAA system should also be designed toprevent the issuance of traffic alerts, avoid capturing the attention of, or otherwise precludingincreases in workload, or prevent additional vectoring requirements for ATC and the UAS pilot.This work attempts to provide guidance for DAA standards for “well clear” (following 14 CFR§91.113) that consider these ATC and UAS pilot concerns. Further information may also be foundin Consiglio et al. (2015).2
Phase I – Air Traffic Controller Acceptability Study1. Phase I General Information1.1Approach and ObjectivesThe primary focus of the Phase I portion of the Collision Avoidance, Self-Separation, and AlertingTimes (CASSAT) experiment was to address minimum and maximum acceptable declarationtimes for projected well clear losses from the perspective of active air traffic controllers. In thissimulation study, controllers managed a mix of manned aircraft and DAA-equipped UAS trafficand provided ratings on acceptability of Horizontal Miss Distances (HMDs) (see section 2.7.1)when near traffic encounters occurred, acceptability of alerting times, and workload ratings duringtest sessions.The following research questions, which drove the experiment design, were proposed:A. Given a projected well clear loss, which of the three values evaluated is the minimumacceptable alert time?B. Given a projected well clear loss, which of the three values evaluated is the maximumacceptable alert time?C. Which, if any, of the alert times are too excessive leading to nuisance alerts for the airtraffic controllers?D. Is there an interaction between Alerting Time and Horizontal Miss Distance?2. Phase I Method2.1SubjectsEleven active Air Traffic Controllers, with no experience at the Dallas-Fort Worth (DFW) Eastside facility, were recruited to perform traffic separation tasks for the scenarios developed in thephase I study. Each of the controllers was the sole controller and performed ATC tasks in thesimulated DFW East-side environment over a span of three days. To maintain a near real-worldenvironment and workload similar to that of actual DFW traffic, background traffic was controlledby pseudo-pilots at two separate pilot stations located in another room within the Air TrafficOperations Laboratory (ATOL, see Appendix A for the layout). UAS aircraft were controlled bytwo additional pseudo-pilots each having access to Ground Control Station (GCS) displaysshowing the self-separation guidance information in real-time; ground control stations werelocated in a third separate room within the ATOL. Pseudo-pilots in this study refer to pilots whowere either part of the research team or were trained and hired by the research team to regularlyparticipate in the CAS studies. ATC subjects were also in communications with other controllersat towers and adjacent airspace who handled handoffs to and from the subject controller’s airspace.These “other” controllers were trained and hired by the research team to regularly participate inthe CAS studies. For a visual representation of the lab layout, please see Appendix A.3
2.2ProcedureThe traffic scenarios were designed so that there were 14 UAS traffic encounters per scenariosession for six hours of evaluation, with each scenario session lasting approximately one hour. Aninitial questionnaire (Appendix B) and training occurred on day one. On day two, additionaltraining runs were conducted followed by the first three hours of testing with the remaining threehours of testing on day three. UAS aircraft were controlled by two pseudo-pilots, each havingaccess to GCS displays showing the DAA self-separation guidance information in real-time.Background traffic, to maintain the environment and workload close to that of actual DFW traffic,was controlled by pseudo-pilots at two additional pilot stations. The controller managed themanned and unmanned traffic which were all flying in the same airspace (DFW East side) andcommunicating with ATC by simulated Very High Frequency (VHF) radio communications.Communications with the UAS were handled the same as manned aircraft communications. Theauditory communications with the UAS had a 400 millisecond (msec) delay each way to simulateterrestrial link and digital link delays. Prior results from the CAS-2 study showed that 400 msecdelays were not a problem for controllers and often not noticed. UAS traffic encounters werebetween the UAS and Visual Flight Rules (VFR) traffic that was transmitting position and altitudeinformation (transponding) but not in voice communications with ATC. This meant that if amaneuver was required to maintain separation, the UAS would make the maneuver. AdditionalIFR and VFR traffic present was traffic taking off, landing, or transitioning the airspace andcommunicating with the controller. Background traffic in the airspace consisted of about 50aircraft per hour which were a mix of IFR and VFR aircraft.2.3Independent VariablesTo address the research questions noted above, multiple independent variable of interest wereexamined, which included:1. Horizontal Miss Distance (HMD). There was an adjustable parameter used in the DAAself-separation algorithms that yields a given HMD if the UAS pilot flies just at the edgeof the navigation display guidance “bands.” See Chamberlain et al. (2015) for details. TheUAS pilots who were part of the research team would fly at the edge of this guidance,yielding the desired HMD for each particular encounter distance, so that controllers couldevaluate that distance. Simulator data yielded the actual Closest Point of Approach (CPA)during the encounter as a check to insure that the desired HMD was obtained. Thecontroller observed the miss distance and geometry on the radar scope and evaluate theacceptability of that HMD.2. Alerting Time. The amount of lead time the guidance presented to the UAS pilot beforeloss of well clear.3. Encounter Geometry. The geometry between the aircraft in the encounter situation and thespeed differentials between the encountering aircraft. The variable of interest for a minorityof encounters (vertical encounters) was a “look-ahead” parameter of Time to Co-altitude(TCOA) that was used by the Detect and Avoid Alerting Logic for Unmanned Systems(DAIDALUS) algorithms.The parameters of these independent variables are shown in Table 1. Additionally, two variablesthat were manipulated in the earlier CAS-1 and CAS-2 studies were held constant for this study.4
These were a fixed wind profile of 21 knots at 3000 feet and a fixed 400 msec voicecommunications delay with the UAS pilot.Table 1 - Independent Variables for Phase IIndependent ValuesNumberValuesHorizontal Miss Distances(HMD)3 values0.7, 1.0, 1.5 Nautical Miles (nmi)Alerting Time (used byDAIDALUS algorithms)3 values30, 45, and 75 secondsEncounter Geometry5 valuesHead-on, Overtake, Crossing, Vertical Overtake,Vertical CrossingTime to Co-altitude (TCOA)for vertical encounters (usedby DAIDALUS algorithms)2 values0 and 20 secondsThe encounter geometries noted in Table 1, had the following specifications. Head-on: intruder(VFR manned) track at 180 degrees from ownship (UAS) /- 15 degrees; Crossing: intruder trackat 90 degrees from
National Aeronautics and Space Administration Langley Research Center Hampton, Virginia 23681-2199 April 2016 NASA-TM-2016-219181 Unmanned Aircraft Systems Human-in-the-Loop