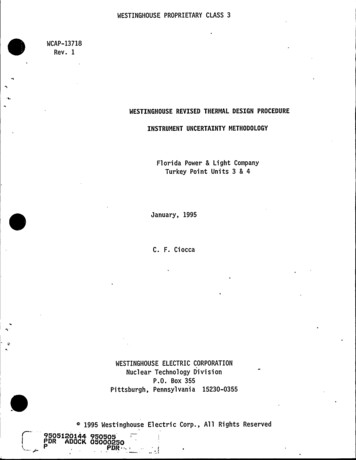
Transcription
WESTINGHOUSE PROPRIETARY CLASS 3WCAP-13718Rev.1WESTINGHOUSE REVISED THERMAL DESIGN PROCEDUREINSTRUMENT UNCERTAINTY METHODOLOGYLight CompanyTurkey Point Units 3 E 4FloridaJanuary,Power81995C. F. CioccaWESTINGHOUSE ELECTRIC CORPORATIONNuclear Technology DivisionP.O. Box 355Pittsburgh, PennsylvaniaP,e 1995 Westinghouse9505 iOZm V50505ADOCK 05000250PDRPDR"- 15230-0355Electric Corp., All RightsReserved
TABLE OF INSTRUMENTATION UNCERTAINTIESIV.CONCLUSIONS26V.REFERENCES27
l'
LISTTable Number OF TABLESTitlePagePressurizer Pressure ControlSystem AccuracyRodControl System AccuracyCalorimetricInstrumentation UncertaintiesFlow4FlowCalorimetric ertainties18Cold Leg Elbow TapFlow Uncertainty21CalorimetricInstrumentation Uncertainties24Secondary Si de Power Cal or imetri cMeasurement Uncertainties25Power
I
LISTFigure NumberOF FIGURESTitleRCSFlowPageCalorimetric SchematicPower Cal orimetri c Schemati c1112930
WESTINGHOUSE REVISED THERMAL DESIGN PROCEDUREINSTRUMENT UNCERTAINTY METHODOLOGY FORTURKEY POINT UNITS 3I.814INTRODUCTIONFour operating parameter uncertainties are used in the uncertainty analysis ofthe Revised Thermal Design Procedure (RTDP). These parameters are PressurizerPressure, Primary Coolant Temperature (T,„,), Reactor Power, and ReactorCoolant System Flow. They are frequently monitored and several are used forcontrol purposes. Reactor power is monitored by the performance of asecondary side heat balance (power calorimetric) once every 24 hours. RCSflow is monitored by the performance of a precision flow calorimetric at thebeginning of each cycle. The RCS Cold Leg elbow taps are evaluated againstthe precision calorimetric and used for monthly surveillance (with a smallincrease in uncertainty). Pressurizer pressure is a controlled parameter andthe uncertainty reflects the control system. T,„, is a controlled parametervia the temperature input to the rod control system and the uncertaintyreflects this control system. This report is based on the elimination of RTDBypass Loops in the design to measure hot and cold leg reactor coolant systemThe RTDP"" is used to predict the plant's DNBR design limit,temperatures.The RTDP methodology considers the uncertainties in the system operating plantparameters, fuel fabrication and nuclear and thermal parameters and includesthe use of various DNB correlations. Use of the RTDP methodology requiresthat variances in the plant operating parameters be justified. The purpose ofthe following evaluation is to define the specific Turkey Point Units 3 5 4Nuclear Plant instrument uncertainties for the four primary system operatingparameters.involved with the development of several techniques totreat instrumentation uncertainties. An early version (for D. C. Cook 2 andTrojan) used the methodology outlined in WCAP-8567 "Improved Thermal DesignProcedure","' which is based on the conservative assumption that theuncertainties can be described with uniform probability distributions.Westinghousehas beenAnother approach (for McGui re and Catawba) is based on the more realisticassumption that the uncertainties can be described with random, normal, twosided probability distributions.approach is used to substantiate the'his
acceptability of the protection system setpoints for many Westinghouse plants,e.g., D. C. Cook 2", V. C. Summer, Wolf Creek, Millstone Unit 3 and others.The second approach is now utilized for the determination of allinstrumentation errors for both RTDP parameters and protection functions.-2-
II.METHODOLOGYerror components forof the squares of those groups ofchannel is thecomponents whichsquare root of the sumare statistically independent. Those errors that are dependent arecombined arithmetically into independent groups, which are thensystematically combined. The uncertainties used are considered to berandom, two sided distributions. The sum of both sides is equal to theThe methodology usedtocombine theafor that parameter, e.g., Rack Drift is typically]"'.j"', the range for this parameter is [This technique has been utilized before as noted above, and has beenand various industry standards'"" .endorsed by the NRC staffrange""relationships between the error components and the channel instrumenterror allowance are variations of the basic Westinghouse SetpointMethodology'" and are defined as follows:Theindication using Special Testdigital volt meter (DVM) at the input to the racks;Equipment orFor precision parameteraCSA ((SCA SMTE SD) (SPE) (STE) (RDOUT))'BIAS2.For parameterCSA 3.Eq.indication utilizing the plant process computer;((SCA SMTE SD) (SPE) (STE)BIAS(RTE) (ID) (A/D) }'For parametersI (RCA RMTE RD)'Eq. 2which have control systems;((PMA) (PEA) (SCA SMTE SD) (RCA RMTE RD CA) (RTE) ) 'CSA (SPE) (STE) BIASEq. 3in equations I and 2 since the equationsare to determine instrumentation uncertainties only. PMA and PEA terms areincluded in the determination of control system uncertainties.PMA andPEAterms are not includedwhere:-3-
CSAChannel AllowancePMARMTEProcess Measurement AccuracyPrimary Element AccuracySensor Calibration AccuracySensor Measurement and Test Equipment AccuracySensor Pressure EffectsSensor Temperature EffectsSensor DriftRack Calibration AccuracyRack Measurement and Test Equipment AccuracyRTERack TemperatureRDRack DriRDOUTReadout Device AccuracyPEASCASMTESPESTESDRCAIDA/DCAThe parametersftor gauge)Isolator DriftAnalog to Digital Conversion AccuracyController Accuracyabove are as definedStandard PMC 20.1, 1973"" .paraphrased below:PEA(DVMComputerSAMAPMAEffectsin referencesHowever,forare based onease in understanding they are5 and12 andnon-instrument related measurement errors, e.g., temperaturestratification of a fluid in a pipe.errors due to a metering devi ce, e.g., elbow, venturi,orifice.SCASPESTESDRCARTEreference (calibration) accuracy for a sensor or transmitter.change in input-output relationship due to a change in staticpressure for a differential pressure (d/p) cell.change in input-output relationship due to a change inambient temperature for a sensor or transmitter.change in input-output relationship over a period of time atreference conditions for a sensor or transmitter.reference (calibration) accuracy for all rack modules in loopor channel assuming the loop or channel is string calibrated,or tuned, to this accuracy.change in input-output relationship due to a change inambient temperature for the rack modules.4-
RDRDOUTIDin input-output relationship over a period of time atreference conditions for the rack modules.the measurement accuracy of a special test local gauge,digital voltmeter or multimeter on it's most accurateapplicable range for the parameter measured.change in input-output relationship over a period of time atreference conditions for a control or protection signalchangeisolating device.allowance for conversion accuracy ofCAan analogsignal toadigital signal for process computer use.allowance for the accuracy of a controller, not includingdeadband.BIAS-anon-randomuncertainty forasensor or transmitter oraprocess parameter.detailed explanation of the Westinghouse methodology noting theinteraction of several parameters is provided in references 5 and 12.A moreIII.INSTRUMENTATION UNCERTAINTIESinstrumentation uncertainties will be discussed first for the twoparameters which are controlled by automatic systems, Pressurizer Pressure,and T,„, (through Rod Control).ThePRESSURIZER PRESSUREPressurizer Pressure is controlled by comparison of the measured vapor spacepressure and a reference value. Allowances are made for the transmitter andthe process racks and controller. As noted on Table 1, the electronics]"'hich corresponds to anuncertainty for this function is []'*'. In addition to the controller accuracy, anaccuracy of [allowance is made for pressure overshoot or undershoot due to the interactionBased on an evaluation of plantand thermal inertia of. the heaters and spray.Therefore, amade for this effect.operation, an allowance of [calculated, which resultstotal control system uncertainty of []"'assuming a normal, two sidedin a standard deviation of []"'asprobability distribution).-5-]"'s
r
TABLEIPRESSURIZER PRESSURE CONTROL SYSTEM ACCURACYSCA MS.TE STE SDBIAS RCA MME RTE RDCAELECTRONICS UNCERTAINTY PLUSELECTRONICS UNCERTAINTY PLUSCONTROLLER UNCERTAINTY-6-
TAYGthe median loop T,„, with areference, derived from the First Stage Turbine Impulse Chamber Pressure. T,„,is the average of the narrow range T„ and T, values. The median loop T,„, isthen used in the controller. Allowances are made (as noted on Table 2) forthe RTDs, transmitter and the process racks and controller. The CSA for thisfunction is dependent on the type of RTD, pressure transmitter, and thelocation of the RTDs, i.e., in the RTD bypass manifold or in the hot and coldlegs. Based on the assumption that two T„ and one T, cross-calibrated WeedRTDs are used to calculate T,„, and the RTDs are located in the hot and coldAssuming a normal, twolegs, the CSA for the electronics is [sided probability distribution results in an electronics standard deviationT,„,is controlledthatby a systemcompares]"'.( )tof [this does not include the controller deadband of 1.5 'F. For T,„,controller accuracy is the combination of the instrumentation accuracy andThe probability distribution for the deadband has beendeadband.However,thethedetermined to be []."'he variance]Combining the varianceforthe deadband uncertainty is then: 4 Cfor instrumentationand deadbandresults inacontroller variance of:]Thecontroller e, [temperature streaming, the]"'nd, with.a C C[total uncertainty is [-7-]"'ias] L,Cfor cold leg
TABLE 2ROD CONTROL SYSTEM ACCURACYSENSOR/TRANSMITTERTurbine PressureTavg a,cPMA SCA SMTE STE SDBIAS PROCESSRACKSTavgERIEAOANALOGTURBINEMSSRCA RMTE RTE tRDCA8 HOT LEG RTDs 28 COLD LEG RTDs IROD CONTROL SYSTEM ACCURACYSENSOR/TRANSMITTERTurbine PressureTavg d,cPMA SCA SMTE STE SDBIAS PROCESSRACKSTavgERIEAOANALOGTURBINERCA RMTE RTE RDCA a,cELECTRONICS CSAELECTRONICS SIGMA CONTROLLER SIGMACONTROLLER BIASCONTROLLER CSA-8-MSS
IRCSFLOWplant Technical Specifications requireflow measurement with aSix month drift effects have been included forhigh degree of accuracy.feedwater temperature, feedwater flow, steam pressure, and pressurizerIt is assumed for this error analysis that the flow measurement ispressure.performed within ninety days of completing the cross-calibration of the hotleg and cold leg narrow range RTDs. Therefore, partial drift effects areincluded. It is also assumed that the calorimetric flow measurement isperformed at the beginning of a cycle, i.e., no allowances have been made forFeedwater venturi fouling, and the calorimetric is performed above 90K RTP.RTDPandflow measurement is performedan RCSdetermining the steam generator thermaloutput (corrected for the RCP heat input and the loop's share of primarysystem heat losses) and the enthalpy rise (Delta-h) of the primary coolant.Assuming that the primary and secondary sides are in equilibrium, the RCStotal vessel flow is the sum of the individual primary loop flows, i.e.,ThebyEq. 4individual primary loop volumetric flows are determined by correcting thethermal output of the steam generator for steam generator blowdown (if notsecured), subtracting the RCP heat addition, adding the loop's share of theprimary side system losses, dividing by the primary side enthalpy rise andmultiplying by the cold leg specific volume. The equation for thiscalculation is:TheWL(A)tQseQp (N)](VgEq. 5(h„- hgwhere;flowW„LoopA0.1247 gpm/(ft'/hr)Steam Generator thermal output (Btu/hr)RCP heat addition (Btu/hr)QsGQp(gpm)
0
heat addition (Btu/hr)Primary system net heat losses (Btu/hr)Specific volume of the cold, leg at T, (ft'/lb)Number of primary side loopsHot leg enthalpy (Btu/lb)Cold Leg enthalpy (Btu/lb).RCPQpQg cNh„hcoutput of the steam generator is determined by a precisionsecondary side calorimetric measurement, which is defined as:The thermalQG where;h, hftW, (h,-h)W,Eq. 6enthalpy (Btu/lb)Feedwater enthal py (Btu/1 b)Feedwater flow (lb/hr).Steamenthalpy is based on the measurement of steam generator outlet Steampressure, assuming saturated conditions. The Feedwater enthalpy is based onThethe measurement of Feedwater temperature and Feedwater pressure.Feedwater flow is determined by multiple measurements and the followingThe Steamcalculation:Eq.coefficientventuri correction for thermal expansionFeedwater venturi flowwhere;FaFeedwaterPrFeedwater densityhPFeedwater venturi pressure(lb/ft')drop (inches H,O).venturi flow coefficient is the product of a number of constantsincluding as-built dimensions of the venturi and calibration tests performedby the vendor. The thermal expansion correction is based on the coefficientof expansion of the venturi material and the difference between FeedwaterFeedwater density is based on thetemperature and calibration temperature.The venturimeasurement of Feedwater temperature and Feedwater pressure.pressure drop is obtained from the output of the differential pressure cellconnected to the venturi.The Feedwatert-7-10-
RCPis determinedheat additioncoolant flow,pumpbyhead, and pumpcalculation, based on the best estimate ofhydraulic efficiency.primary system net heat losses are determined by calculation, consideringthe following system heat inputs and heat losses:TheCharging flowLetdownflowinjection flowthermal barrier cooler heatSealRCPremovalPressurizer spray flowPressurizer surge line flowComponentinsulation heat lossessupport heat lossesheat losses.ComponentCRDMsingle calculatedheat inputs.Asumfor100% RTPoperation isusedfor these losses orleg and cold leg enthalpies are based on the measurement of the hotleg temperature, cold leg temperature and the Pressurizer pressure. The coldleg specific volume is based on measurement of the cold leg temperature andPressurizer pressure.The hotTheRCSflow measurement is thusbased onthe following plant measurements:Steamline pressure (P,)Feedwater temperature (Tf)Feedwaterventuri differential pressureHot leg temperatureCold Leg temperature(T„)(Tq)Pressurizer pressure (Pp)Steam Generator blowdownand on the(if notsecured)following calculated values:*Feedwaterventuri flow coefficients (K)-11-(hP)
FeedwaterFeedwaterFeedwaterFeedwaterventuri thermal expansion correction (F,)density (p,)enthalpy (hf)pressure (P,)Steam enthalpy (h,)Moisture carryover (impacts h,)Primary system net heat losses (g,)RCP heat addition ((}p)Hot leg enthalpy (h„)Cold leg enthalpy (h,).These measurementsTheandderivation of thecalculations are presented schematicallyonFigureerrors and flow uncertaintiesonTablemeasurementl.5arenoted below.eSecondary Sideside uncertainties are in four principal areas, Feedwater flow;Feedwater enthalpy, Steam enthalpy and RCP heat addition. These four areasThe secondaryarespecifically identifiedonTable 5.the measurement of Feedwater flow, each Feedwater venturi was calibratedby the vendor in a hydraulics laboratory under controlled conditions to anThe cal ibrati on data whi ch substanti ates thi saccuracy of []accuracy i s pr ovi ded to the plant by the vendor. An'dditional uncertaintyincluded -for installation effects, resulting in afactor of [conservative overall flow coefficient (K) uncertainty ofRCS loop flow is proportional to steam generator][thermal output which is proportional to Feedwater flow, the flow coefficientshould be noted that nouncertainty is expressed as []allowance is made for venturi fouling. The venturis are inspected, andIfcleanednecessary, prior to performance of the precision measurement.fouling is present but not removed, its effects must be treated as a flowFor.""]"'s."'ince."'tifTheuncertainty applied to the Feedwater venturi thermal expansion correction-12-
is based on the uncertainties of the measured Feedwater temperature andthe coefficient of thermal expansion for the venturi material, usually 304stainless steel. For this material, a change of l 'F in the nominal( F,)Feedwater temperaturerange changesthermal output by the same amount.the steam generatorF, by a0.002% anduncertainty in F, of t5X for 304 stainless steel is used in this analysis.Feedwater flow.This results in an additional uncertainty of [Westinghouse uses the conservative value of []An]"'n."'singitis possible to determine the sensitivitiesthe 1967 ASME Steam Tablesof various parameters to changes in Feedwater temperature and pressure. Table3 notes the instrument uncertainties for the hardware used to perform theTable 4 lists the various sensitivities. As can be seen onmeasurements.Table 4, Feedwater temperature uncertainties have an impact on venturi F„Feedwater pressure uncertaintiesFeedwater density and Feedwater enthalpy.impact Feedwater density and Feedwater enthalpy.Feedwater venturi hPuncertainties are converted toXFeedwater flow using thefollowing conversion factor:Xflow terflow transmitterspan]"'fis [nominalflow.it ispossible to determine thesensitivity of Steam enthalpy to changes in Steam pressure and Steam quality.Table 3 notes the uncertainty in Steam pressure and Table 4 provides thesensitivity. For Steam quality, the Steam Tables were used to determine thevalue is noted onsensitivity at a moisture content of []Using the 1967ASMESteam Tablesagain,."'hisTable 4.tuncertainty is derived from the combination of the primarysystem net heat losses and pump heat addition. These are summarized .for athree loop plant as follows:The net pump heat-13-
- 2.0System heat lossesMWtComponent conduction and- 1.4convection lossesPump heat adderNet HeatTheuncertaintyon systeminput to 11.4 8 MWtRCSheat losses,is essentially all due totheestimated to be [which]"'fcharging and letdown flows, has beencalculated value. Since direct measurements are not possible, the uncertaintyon component conduction and convection losses has been assumed to bethe calculated value. Reactor coolant pump hydraulics are known[to a relatively high confidence level, supported by system hydraulics testsperformed at Prairie Island II and by input power measurements from severalplants, therefore, the uncertainty for the pump heat addition is estimated tothe best estimate value. Considering these parameters as onebe [quantity, which is designated the net pump heat uncertainty, the combineduncertainties are less than the value used in the analysis, which is]"'f]"'f[]"'fcore power.Primary Sideuncertainties are in three principal areas, hot leg enthalpy,cold leg enthalpy and cold leg specific volume. These are specifically notedon Table 5. Three primary side parameters are actually measured, T„, T, andPressurizer pressure. Hot leg enthalpy is influenced by T„, Pressurizerpressure and hot leg temperature streaming. The uncertainties for theinstrumentation are noted on Table 3 and the sensitivities are provided onTable 4. The hot leg streaming is split into random and bias (systematic)For Turkey Point Units 3 5 4, the RTDs are located in thermowellscomponents.placed in the loops (bypass manifolds eliminated) . A plant specificevaluation has been performed which resulted in a streaming uncertainty ofsystematic components.random and [[The primary side]"'or]"'or1The cold leg enthalpy anduncertainties are impacted by T, andnotes the T, instrument uncertainty and Table 4specificPressurizer pressure. Tableprovides the sensitivities.3volume
Noted on Table 5is the plant specificallowance.necessary,Whenerror due to thefor this plant.RTDRTDcross-calibration systematicallowance is madecross-calibration procedure.anforNoasystematic temperatureallowance was necessaryParameter dependent effects are identified on Table 5. Westinghouse hasdetermined the dependent sets in the calculation and the direction ofinteraction, i.e., whether components in a dependent set are additive orsubtractive with respect to a conservative calculation of RCS flow. The sameAs a result, thework was performed for the instrument bias values.calculation explicitly accounts for dependent effects and biases with credittaken for sign (or direction of impact).Using Table 5, the3loop uncertainty equation (with biases)isasfollows:0the number of loops, number, typethe vessel Delta-T, the flow is:Based on8of loopsflow uncertainty[] t,c-15-and measurement(Xflow)methodofRTDs, and
TABLE 3FLOW CALORIMETRIC INSTRUMENTATION UNCERTAINTIESFW TEMP(X SPAN)FW PRESFWhPSTM PRESSTHTCPRZ PRESSSCASMTE SPESTESDRCARMTE RTERDRDOUT BIAS CSA¹OF INST USEDFINST SPAN PSIX hPPSIoFoFPSIjul[CINST UNC.(RANDOM) INST UNC.(BIAS)NOMINAL 437'F885PSIA785 PSIA-16-602.3'F546.2'F2250 PSIA
TABLE 4FLOW CALORIMETRICSENSITIVITIESFEEDWATER EDELTAPFEEDWATER ENTHALPYTEMPERATUREPRESSURE 1199.8 BTU/LBM415.5 BTU/LBM784.3 BTU/LBM 616.5 BTU/LBM542.5 BTU/LBM74.1 BTU/LBM 1. 440 BTU/LBM-DEGFhShFDh(SG)STEAM ENTHALPYPRESSUREMOISTUREHOT LEG ENTHALPYTEMPERATUREPRESSUREhHhCDh(VESS)Cp (TH)COLD LEG ENTHALPYTEMPERATUREPRESSURECp(TC) 1.230 BTU/LBM-DEGFCOLD LEG SPECIFIC VOLUMETEMPERATUREPRESSURE-17-
TABLE 5CALORIMETRIC RCS FLOW MEASUREMENT UNCERTAINTIESINSTRUMENT ERRORCOMPONENTFLOW UNCERTAINTYFEEDWATER FLOWVENTURITHERMAL EXPANSION ESSUREDELTAPFEEDWATER ENTHALPYTEMPERATUREPRESSURESTEAM ENTHALPYPRESSUREMOISTURENET PUMP HEAT ADDITIONHOT LEG ENTHALPYTEMPERATURESTREAMING, RANDOMSTREAMING, SYSTEMATICPRESSURECOLD LEG ENTHALPYTEMPERATUREPRESSURECOLD LEGSPECIFIC VOLUMETEMPERATUREPRESSURE*,**, , INDICATE SETS-18-OF DEPENDENT PARAMETERS
'0
TABLE 5 (CONTINUED)CALORIMETRIC RCS FLOW MEASUREMENT UNCERTAINTIESFLOWUNCERTAINTYBIAS VALUESFEEDWATER PRESSUREDENSITYSTEAM PRESSUREPRESSURIZER PRESSUREENTHALPYENTHALPYENTHALPYENTHALPYFLOW-HOT LEGCOLD LEGSPECIFIC VOLUMECOLD LEG-BIAS TOTAL VALUESINGLE LOOP UNCERTAINTY (WITHOUT BIAS VALUES)3LOOP UNCERTAINTY(WITHOUT BIAS VALUES)-19-
the reference fordetermining the accuracy of the cold leg elbow taps. Since the elbow tap hPis a simple matter to performtransmitters feed the plant process computer,Technical Specification required surveillance. Table 6 notes the instrumentuncertainties for determining flow by using the elbow taps, assuming one elbowtap per loop. The hP transmitter uncertainties are converted to percent flowThe elbow tap uncertainty ison the same basis as the Feedwater venturi bP.then combined with the precision flow calorimetric uncertainty. Thiscombination of uncertainties results in the following total flow uncertainty:Asnotedearlier, the precision flow calorimetric isitkof loops3Theflow uncertaintyof loops3flow) 3;4corresponding values used in0(%RTDPare:standard deviation[j 4C0-20-(Xflow)used as
r0
TABLE 6COLD LEG ELBOW TAP FLOW UNCERTAINTYINDICATED RCS FLOW INPUT VALUES CALL VALUES IN XPEASCASMTE SPEd/pSPANSTESDBIAS RCARMTE RTERDA/0ROUT 8 OF LOOP 3FLOW CALORIMETRIC FLOW CAL. BIASPRESS. CONTROLTEMP. CONTROLFLOW SPANACCURACY OF INDICATED RCS FLOW FROM PROCESSALL VALUES INPMA'XCOMPUTERFLOWPEASCASMTE SPESTESDBIAS RCARMTE RTERDA/0ROUT LOOP ELBOW TAPN LOOP ELBOW TAP1N LOOP RCSFLOW (NO3.4BIAS)-21-X FLOW
primary/secondary side heat balance once every 24hours when power is above 15Ã Rated Thermal Power. This heat balance is usedto verify that the plant is operating within the limits of the OperatingLicense and to adjust the Power Range Neutron Flux channels when thedifference between the NIS and the heat balance is greater than that requiredby the plant Technical Specifications.Generallyaplant performsasides are in equilibrium; the corepower is determined by summing the thermal output of the steam generators,correcting the total secondary power for steam generator blowdown (if notsecured), subtracting the RCP heat addition, adding the primary side systemlosses, and dividing by the core rated Btu/hr at full power. The equation forAssumingthat the primaryand secondarythis calculation is:(Njf@SG—Q ()] (100)Eq. 8where;RPCore power (X RTP)NNumberQsaSteam Generator thermalQpHof primary side loopsoutput (BTU/hr) as defined inRCP heat adder (Btu/hr) as defined in Eq. 5Primary system net heat losses (Btu/hr) as defined inCore rated Btu/hr at full power.Eq. 6Eq. 5the purposes of this uncertainty analysis (and based on H noted above)is assumed that the plant is at 100% RTP when the measurement is taken.Measurements performed at lower power levels will result in differentuncertainty values. However, operation at lower power levels results inincreased margin to DNB far in excess of any margin losses due to increasedmeasurement uncertainty.ForeThe secondaryside power calorimetric equations and effects are the-22-same asit
those noted for the precision flow calorimetric (secondary side portion),equations 6 and 7. The measurements and calculations are presentedschematically on Figure 2. Table 7 provides the instrument uncertainties foris necessary to make thisthose measurements performed. Sincehas been assumed that the plant computer will be useddetermination daily,for the calculations. The sensitivities calculated are the same as thosenoted for the secondary side on Table 4. As noted on Table 8, Westinghousehas determined the dependent sets in the calculation and the direction ofinteraction. This is the same as that performed for the RCS flowcalorimetric, but applicable only to power. The same was performed for thebias values noted. It should be noted that Westinghouse does not include anyititforallowanceinaneffect of fouling is to resulthigher than actual, which is conservative.Feedwater venturiindicated powerfouling.TheUsing the power uncertainty values noted on Table 8, the3loop uncertainty(with bias values) equation is as follows:the number of loops and the instrument uncertainties for the fourparameters, the power measurement uncertainty for the secondary side powerBased oncalorimetric is:8of loopspower3[uncertainty] ITIC-23-(X RTP)
TABLE 7POWER CALORIMETRIC INSTRUMENTATION(X SPAN)FWTEMPFW PRESFW DPUNCERTAINTIESSTM PRESSSCA SMTE SPE STE SDBIAS RCA RMTE RTE RDIDA/D CSA DEG FINST SPAN INSTPSIPSIrUNC(RANDOM) INSTUNC(BIAS)NOMINAL 437'F785 PSIA885 PSIA-24-
TABLE 8SECONDARY SIDE POWER CALORIMETRIC MEASUREMENT UNCERTAINTIESINSTRUMENT ERRORCOMPONENT.,FEEDWATER FLOWVENTURITHERMAL EXPANSION ESSUREDELTAPFEEDWATER ENTHALPYTEMPERATUREPRESSURESTEAM ENTHALPYPRESSUREMOISTURENET PUMP HEAT ADDITIONBIAS VALUESFEEDWATER DELTA PFEEDWATER PRESSUREDENSITYSTEAM PRESSUREENTHALPYENTHALPYPOWERBIAS TOTAL VALUE*,**INDICATE SETS OF DEPENDENT PARAMETERSSINGLE LOOP UNCERTAINTY (WITHOUT BIAS VALUES)3LOOP UNCERTAINTY(WITHOUT BIAS VALUES)-25-POWER UNCERTAINTY
IV.CONCLUSIONSsections provide the methodology to account for pressure,temperature, power and RCS flow uncertainties for the RTDP analysis.The plant specific instrumentation data and procedures supplied by FloridaPower 8 Light Company have been reviewed and the uncertainty calculationscompleted using this data.The precedingi-26-
v.REFERENCESWestinghouse letter NS-CE-1583, C. Eicheldinger to J. F. Stolz,dated 10/25/77.2.letterWestinghouseNS-PLC-5111, T. M. AndersontoE. Case,NRC,NRC,dated5/30/78.3.Westinghouse letter NS-TMA-1837, T. M. Anderson to S. Varga,dated 6/23/78.4.Westinghouse letter NS-EPR-2577,NRC, dated 3/31/82.5.WestinghouseLetterE. P. RaheJr. toNS-TMA-1835, T. M. AndersontoC. H.NRC,Berlinger,E. Case,NRC,dated6/22/78.6.NRCletter,Company,7.S. A. Vargato J. Dolan, Indianaand MichiganElectricdated 2/12/81.operation of VirgilSafety Evaluation Report related to theSummer Nuclear Station Unit No. 1, DocketNo. 4,NUREG-0717 SupplementC.50-395, August, 1982.8.Regulatory Guide 1.105 Rev. 2, "Instrument SetpointsSafety-Related Systems", dated 2/86.9NUREG/CR-3659(PNL-4973), "A Mathematical Model for Assessing theUncertainties of Instrumentation Measurements for Power and Flow ofPWR10.Reactors", 2/85.ANSI/ANS Standardforll.for58.4-1979,"Criteria for Technical SpecificationsNuclear Power Stations".ISA Standard S67.04,1987, r Nuclear Safety-RelatedPlants".
n.Tuley, C. R., Miller, R. B., "Westinghouse Setpoint Methodology forControl and Protection Systems", IEEE Transactions on Nuclear Science,February, 1986, Vol. NS-33 No. 1, pp. 684-687.13.14ScientificApparatus Manufacturers Association, Standard1973, "Process Measurement and Control Terminology".'estinghouseApril,PMC20. 1,WCAP-11397-P-A, "Revised Thermal Design Procedure",1989.-28-dated
V
PRIMARY SIDESECONDARY SIDEP,Pfhsh,PpPfF,W, gQsG- calculated-measuredw„Other LoopsRCSFLOWFigure IRCSFlowCalorimetric Schematic-29-
1I1 J
SECONDARYSIDEF,WqQsaOther LoopsCore PowerPowerfigure 2Calorimetric Schematic-30-
A
WESTINGHOUSE REVISED THERMAL DESIGN PROCEDURE INSTRUMENT UNCERTAINTY METHODOLOGY FOR TURKEY POINT UNITS 3 81 4 I. INTRODUCTION Four operating parameter uncertainties are used in the uncertainty analysis of the Revised Thermal Design Procedure (RTDP). These parameters are Pressurizer Pressure, Primary Coolant Temperature (T,„,), Reactor Power, and Reactor Coolant System Flow. They are .