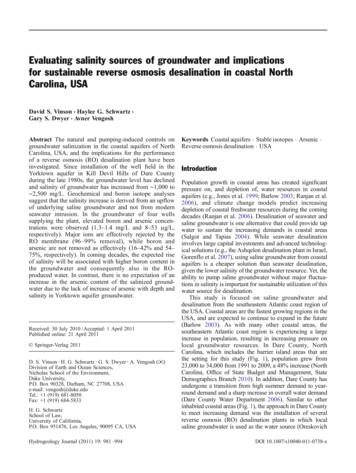
Transcription
Evaluating salinity sources of groundwater and implicationsfor sustainable reverse osmosis desalination in coastal NorthCarolina, USADavid S. Vinson & Haylee G. Schwartz &Gary S. Dwyer & Avner VengoshAbstract The natural and pumping-induced controls ongroundwater salinization in the coastal aquifers of NorthCarolina, USA, and the implications for the performanceof a reverse osmosis (RO) desalination plant have beeninvestigated. Since installation of the well field in theYorktown aquifer in Kill Devil Hills of Dare Countyduring the late 1980s, the groundwater level has declinedand salinity of groundwater has increased from 1,000 to 2,500 mg/L. Geochemical and boron isotope analysessuggest that the salinity increase is derived from an upflowof underlying saline groundwater and not from modernseawater intrusion. In the groundwater of four wellssupplying the plant, elevated boron and arsenic concentrations were observed (1.3–1.4 mg/L and 8–53 μg/L,respectively). Major ions are effectively rejected by theRO membrane (96–99% removal), while boron andarsenic are not removed as effectively (16–42% and 54–75%, respectively). In coming decades, the expected riseof salinity will be associated with higher boron content inthe groundwater and consequently also in the ROproduced water. In contrast, there is no expectation of anincrease in the arsenic content of the salinized groundwater due to the lack of increase of arsenic with depth andsalinity in Yorktown aquifer groundwater.Received: 30 July 2010 / Accepted: 1 April 2011Published online: 21 April 2011* Springer-Verlag 2011D. S. Vinson : H. G. Schwartz : G. S. Dwyer : A. Vengosh ())Division of Earth and Ocean Sciences,Nicholas School of the Environment,Duke University,P.O. Box 90328, Durham, NC 27708, USAe-mail: vengosh@duke.eduTel.: 1 (919) 681-8050Fax: 1 (919) 684-5833H. G. SchwartzSchool of Law,University of California,P.O. Box 951476, Los Angeles, 90095 CA, USAHydrogeology Journal (2011) 19: 981–994Keywords Coastal aquifers . Stable isotopes . Arsenic .Reverse osmosis desalination . USAIntroductionPopulation growth in coastal areas has created significantpressure on, and depletion of, water resources in coastalaquifers (e.g., Jones et al. 1999; Barlow 2003; Ranjan et al.2006), and climate change models predict increasingdepletion of coastal freshwater resources during the comingdecades (Ranjan et al. 2006). Desalination of seawater andsaline groundwater is one alternative that could provide tapwater to sustain the increasing demands in coastal areas(Salgot and Tapias 2004). While seawater desalinationinvolves large capital investments and advanced technological solutions (e.g., the Ashqelon desalination plant in Israel,Gorenflo et al. 2007), using saline groundwater from coastalaquifers is a cheaper solution than seawater desalination,given the lower salinity of the groundwater resource. Yet, theability to pump saline groundwater without major fluctuations in salinity is important for sustainable utilization of thiswater source for desalination.This study is focused on saline groundwater anddesalination from the southeastern Atlantic coast region ofthe USA. Coastal areas are the fastest growing regions in theUSA, and are expected to continue to expand in the future(Barlow 2003). As with many other coastal areas, thesoutheastern Atlantic coast region is experiencing a largeincrease in population, resulting in increasing pressure onlocal groundwater resources. In Dare County, NorthCarolina, which includes the barrier island areas that arethe setting for this study (Fig. 1), population grew from23,000 to 34,000 from 1991 to 2009, a 48% increase (NorthCarolina, Office of State Budget and Management, StateDemographics Branch 2010). In addition, Dare County hasundergone a transition from high summer demand to yearround demand and a sharp increase in overall water demand(Dare County Water Department 2006). Similar to otherinhabited coastal areas (Fig. 1), the approach in Dare Countyto meet increasing demand was the installation of severalreverse osmosis (RO) desalination plants in which localsaline groundwater is used as the water source (OreskovichDOI 10.1007/s10040-011-0738-x
982Fig. 1 Map showing locations and design capacities (m3/day) of RO desalination plants along the Atlantic coast. Symbol size isproportional to total dissolved solids (TDS) of feed groundwater to the RO plants. Data from the American Membrane TechnologyAssociation (AMTA 2011)and Watson 2003; Dare County Water Department 2006).The hydrogeologic properties of the Atlantic coast (Meisler1989; Barlow 2003) enable water systems such as DareCounty’s to pump saline groundwater for the ROdesalination plant instead of the more costly option ofseawater desalination to replace limited supplies ofHydrogeology Journal (2011) 19: 981–994surface water and fresh, shallow groundwater (Fig. 1).Currently, the Dare County Water Department operatesthree small desalination plants with production rates of230–7,570 m3/day (0.08–2.76 106 m3/year). The DareCounty North RO Water Plant at Kill Devil Hills is thefocus of this study, and generates 3,780 m3/day permeateDOI 10.1007/s10040-011-0738-x
983(treated) water that is blended with raw well water toproduce 4,055 m3/day (about 1.5 106 m3/year).Desalination of brackish and/or saline groundwaterpresents other challenges such as the presence of naturallyoccurring contaminants in groundwater that are not removedeffectively by RO desalination, including boron (USEnvironmental Protection Agency (EPA) 2008; Kloppmannet al. 2008) and uncharged forms of arsenic (Oreskovich andWatson 2003; Pawlak et al. 2006; George et al. 2006; Mooreet al. 2008; Walker et al. 2008; Geucke et al. 2009). Highboron concentrations are commonly observed in coastalaquifers due to boron desorption from marine sediments(e.g., D’Avino and Spandre 1995; Vengosh and Spivack1999) and at near-neutral pH, boron occurs primarily asuncharged B(OH)30, which is not efficiently adsorbed and canaccumulate in groundwater. Although saline waters maycontain high arsenic concentrations (Smedley and Kinniburgh2002; Scanlon et al. 2009), high-As groundwater is commonly understood as a function of the solid-phase arsenicsource and desorption processes that are sensitive to pH,redox conditions, and/or surface charge modification bycations (Smedley and Kinniburgh 2002; Haque et al. 2008;Scanlon et al. 2009). At near-neutral pH, oxidized As(V)occurs as anionic H2AsO4– or HAsO42–, subject to pHsensitive desorption from metal oxides; reduced As(III)exists primarily as uncharged H3AsO30 that is lesseffectively adsorbed than anionic As(V). Redox processessubsequent to Fe and Mn oxide reduction such as sulfatereduction, may further affect As mobility in anoxicaquifers (e.g., Kirk et al. 2004).In addition to the potential water-quality problemsintroduced by natural contaminants, the stability of thequality of the saline-water source for the RO plant is inquestion, particularly for aquifers that are constantly beingexploited beyond natural replenishment. This paper aimsto investigate these two issues. First, water-qualitychanges that have occurred since the installation of thepumping wells for the RO desalination plant in DareCounty during the late 1980s are investigated. Majorelements coupled with stable oxygen, hydrogen, andboron isotopes are used as indicators for tracing theorigin of the saline groundwater. Second, the relationshipbetween the chemical composition of the saline groundwaterand the rejection performance, particularly of boron andarsenic, by RO desalination in the Dare County NorthReverse Osmosis Water Plant is assessed. In addition,predictions are made on how the long-term changes in thewater quality of the saline groundwater source will affect thequality of the produced water generated by the RO plant. Thecase study of Dare County in North Carolina is representative of numerous areas along the Atlantic coast that arefacing similar challenges for sustainable water supply underincreasing water demands (Fig. 1).Hydrogeologic settingThe Atlantic Coastal Plain extends from Florida to NewYork and is comprised of thick clastic sediments andHydrogeology Journal (2011) 19: 981–994marine limestones from Jurassic to Quaternary in age. InNorth Carolina, the area of this research, the Coastal Plainmay be divided into nine distinct sand and limestoneaquifers (Fig. 2a–b). Across a gently dipping outcrop beltabout 200 km wide, these sediments reach a thickness ofapproximately 3,000 m (Winner and Coble 1996). In theaquifers of the Atlantic Coastal Plain, waters movingdown-gradient undergo ion exchange reactions thatremove Ca and Mg; thus, in the down-gradient, confinedportions of the aquifers, Na-bicarbonate waters dominate(Chapelle and Knobel 1983; Knobel et al. 1998) beforemixing with saline Na–Cl waters. Overall, physical andgeochemical characteristics suggest that this saline sourcein the deep confined Coastal Plain aquifers is dilute fossilseawater and/or brine and that the salinity level of thiswater is derived from mixing caused by Quaternary sealevel fluctuations (Manheim and Horn 1968; Meisler1989; Barlow 2003). No confining unit separates theaquifer from the natural salinity source down-dip,whereas regional confining unit(s) separate the aquifersystem from modern seawater. Therefore, intrusion ofmodern seawater is not suspected as a significant causeof the groundwater’s salinity. Although the authorsknow of no isotopic measurements of the confinedcoastal aquifers of eastern North Carolina (e.g., 14C)that would directly address the antiquity of this salinewater source, 14C dating of inland waters in confinedCretaceous Coastal Plain aquifers at similar depths asthis study indicates long groundwater residence timesince recharge (3,200–26,000 years; Kennedy andGenereux 2007). Therefore, surface-derived inputs tothese confined aquifers are not substantial. Redoxconditions in the confined Coastal Plain aquifers aregenerally anoxic and sulfate-reducing (Chapelle andMcMahon 1991; Knobel et al. 1998; Kennedy andGenereux 2007).The Yorktown aquifer, in which the study wells arelocated, is an upper Miocene to Pliocene marine sandcontaining abundant silt, clay, and carbonate shell materialand is the uppermost confined aquifer in the AtlanticCoastal Plain (Fig. 2d; Winner and Coble 1996). Thewestern, relatively shallow section of the Yorktownaquifer receives sufficient recharge from the surface thatonly localized water level declines have been observed(dePaul et al. 2008). However, the eastern one third of theYorktown aquifer in North Carolina, including the studyarea, exhibits higher hydraulic head than overlyingaquifers as is generally true in the confined Coastal Plainaquifers (Winner and Coble 1996). Like other CoastalPlain aquifers, the Yorktown aquifer becomes thicker andmore saline down-dip towards the Atlantic Ocean; thepoint at which Cl 250 mg/L at the base of the Yorktownaquifer lies approximately 60 km inland of the study area(Lautier 2009). In the vicinity of the study site, theYorktown aquifer extends from a depth of 50 m to atleast 200 m below sea level and is overlain by a silt- andclay-rich confining unit up to 15 m thick and by theQuaternary surficial aquifer (Fig. 2c–d; Winner and Coble1996).DOI 10.1007/s10040-011-0738-x
984acdbFig. 2 Map and cross sections showing location of the study site and depths of wells sampled: a Geologic map based on North CarolinaGeological Survey (1985) showing locations of wells J3O3, J7K5, and J7K7; b Schematic cross section of the North Carolina Coastal Plainmodified from Winner and Coble (1996; plate 7); c Inset map showing location of Kill Devil Hills well field, where all other wells weresampled; and d Schematic diagram indicating approximate depths of wells sampled within the Yorktown aquifer (interval labeledProduction Zone indicates the screen length of high-capacity production wells)Hydrogeology Journal (2011) 19: 981–994DOI 10.1007/s10040-011-0738-x
985Materials and methodsResults and discussionWater samples were collected from four productionwells, screened at approximately 90–120 m depth; threenested monitoring wells adjacent to a production well(depth 95–190 m; Fig. 2d); two coastal monitoringwells seaward from the production well field (depth 120 m); and seven points within the RO desalinationplant at Kill Devil Hills, Dare County, North Carolina(Table 1). In addition to the wells in the Kill Devil Hillsarea, three monitoring wells (depth 24–67 m) on themainland and near the town of Manteo on RoanokeIsland (Fig. 2a) were sampled at sites maintained by theNorth Carolina Division of Water Resources. Thesethree wells are shallower than the wells at Kill DevilHills, chosen to represent shallow recharging watersaway from possible marine sources, and thus may notrepresent the same stratigraphic level of the Yorktownaquifer. Monitoring wells were sampled after a periodof continuous pumping to remove stagnant water. pH,electrical conductivity, dissolved oxygen, and temperature were measured in water samples on site (Table 1).The samples were returned to Duke University for theanalysis of major and trace elements coupled withboron and stable oxygen and hydrogen isotopes.Concentrations of B, As, and Sr were determined byinductively coupled plasma-mass spectrometry calibrated using NIST 1643e trace element solution.Arsenic concentrations were corrected for chlorideinterference by the equivalent analyte concentrationmethod (Taylor 2001). Ca, Mg, and Na concentrationswere determined by direct current plasma spectrometryand potassium concentration was determined by flameatomic absorption spectrometry. Anion (chloride, sulfate, andbromide) concentrations were measured by ion chromatography. Effective detection limits and analytical precision varyby dilution factors (B 0.2–10 μg/L, As 0.01–0.6 μg/L).Bicarbonate concentrations were determined by titration topH 4.5. Oxygen and hydrogen isotope ratios were determinedin the Duke Environmental Isotope Laboratory by injectionof 1 μL samples from a gas-tight syringe into aThermoFinnigan thermochemical analyzer, using H2and CO gas peaks analyzed for isotope ratios with aThermoFinnigan Delta XL isotope ratio mass spectrometerand normalized against V-SMOW and V-SLAP. Precision isapproximately 0.3‰ for δ2H and 0.1‰ for δ18O. Boronisotope ratios were determined by negative ion thermalionization mass spectrometry using a Thermo ScientificTRITON thermal ionization mass spectrometer at DukeUniversity. Filtered water samples were treated by H2O2oxidation, then 2 ng of B was loaded onto outgassedrhenium filaments in a synthetic seawater solution containing Na, Mg, Ca, and K from high-purity single-elementstandard solutions in a laminar-flow hood with B-freefilters (Dwyer and Vengosh 2008). Ratios are normalized tothe NIST 951 standard and reported as δ11B. Repeatedanalysis of NIST 951 and seawater yielded average 11B/10Bof 4.0058 (1σ 0.0011 or 0.3‰, n 62) and 4.1630 (1σ 0.0025, n 17; δ11B 39.2 0.6‰), respectively.Salinization of groundwater in the Yorktown aquiferHydrogeology Journal (2011) 19: 981–994Groundwater from shallow monitoring wells exhibitsrelatively low salinity relative to the deep monitoringwells with chloride content of 8,200–9,900 mg/L(Fig. 3; Table 1). The Dare County RO desalinationplant uses saline groundwater from several productionwells at intermediate depth (Fig. 2d, Table 1). Since theinstallation of these production wells, a total volume of70 106 m3 has been extracted with a pumping rate inthe range of 0.2 – 0.5 106 m3/year per well. Operationof the production wells since the late 1980s hascoincided with a general decline in the water table asmeasured in the monitoring wells (Fig. 4a): from adepth of 10–12 m in the early 1990s to a depth range of13–16 m during the late 2000s. This suggests thatpumping rates have exceeded the natural replenishmentof this aquifer. The salinity in these wells has alsoincreased since the installation of the pumping. Historicdata for the production wells show that the chloridecontent has increased from an initial concentration of970 mg/L in August 1989 to approximately 2,500 mg/Lby 2009 (Fig. 4). During the first five years of pumping,the salinity increase rate was high ( 190 mg/L peryear), followed by a lower salinization rate ( 45 mg/Lper year), indicated by the leveling of the chlorideconcentration trend (Fig. 4b).Tracing the source of the salinity by geochemicaland isotope techniquesIn order to evaluate the origin of salinization of theproduction wells, the geochemical composition of salinegroundwater at different depths in the Yorktown aquiferwas investigated (Table 1). First, ion ratios, δ2H, and δ18Oare consistent with mixing between shallow freshwaterand deep saline water. Mixing with upcoming saline wateris supported by (1) variations of δ2H and δ18O (Fig. 5)that indicate a mixing relationship between seawater-likesaline groundwater (δ2H and δ18O here approximated at0‰ although analysis of North Carolina coastal surfacewater indicates median δ18O of 0.92‰; n 40; Schmidtet al. 1999) and low-salinity groundwater derived fromlocal recharge (δ2H 20‰; δ18O 4.5‰; Table 2);and (2) a constant Br/Cl ratio near the seawater value of1.5 10–3 (Fig. 6). Water from the production zone wells isconsistent with mixing between the deep and shallowgroundwater, based on Br/Cl, Na/Cl, Mg/Cl, Ca/Cl, andSr/Cl ratios, although these ion ratios are somewhatmodified by cation exchange. For a hypothetical verticalmixture between wells 1–310 and 1–610, ion ratiosconsistently indicate that inputs of 20–25% deep salinewater can explain the chemistry of produced water(Fig. 6).Secondly, the nature of the deep saline water thatcontributes to the Kill Devil Hills well field wasexamined. Evaluating the source of the saline component(e.g., seawater, old modified seawater, brackish water, orDOI 10.1007/s10040-011-0738-x
Screen depth (m)DatepHT ( C)Hydrogeology Journal (2011) 19: 74744.53.718424.9K(mg/L)baND indicates not detected. Detection limit depends on dilution factor but in all cases ND indicates As 1 μg/L. These are plotted as 0 μg/LWells 1–310, production well 1, 1–510, and 1–610 are a nest at the same locationcData from Millero and Sohn (1991), except B concentration from Vengosh and Spivack (1999)Mainland and Roanoke Island monitoring wells (NC Division of Water Resources)24–2711 Dec 20086.817.8J7K793–9611 Dec 20087.918.5J7K561–6711 Dec 20087.217.5J3O3Kill Devil Hills coastal monitoring wells 12021 Nov 20088.6Ocean Bay 12021 Nov 20088.018.8AlbatrossKill Devil Hills well fieldb9521 Nov 20088.01-310 90–12028 Oct 20087.920.0Production well 115628 Apr 20097.221.41-51018728 Apr 20097.221.41-610 90–12029 Oct 20087.820.0Production well 2 90–12021 Nov 20087.819.5Production well 7 90–12021 Nov 20087.8Production well 11Reverse osmosis facility29 Oct 20087.820.0D1 (raw water blend)29 Oct 20087.920.7D3 (1st stage brine)29 Oct 20086.920.2D4 (2nd stage permeate)29 Oct 20087.821.3D5 (2nd stage brine)29 Oct 20087.920.9D6 (combined permeate)29 Oct 20088.420.2D7 (drinking water)29 Oct 20087.720.5D8 (final brine)c8.2SeawaterSample CO3–(mg/L)Table 1 Field parameters, major and trace elements for groundwater and reverse osmosis plant waters. Steps in the reverse osmosis process are detailed in Figure .1515433NDND515381.918ND125NDAsa(μg/L)986DOI 10.1007/s10040-011-0738-x
0987100200150Depth (m)50Mainland & Roanoke IslandShallow monitoring wellsProduction wellsDeep monitoring wells025005000750010000Cl (mg/L)Fig. 3 Relationship between chloride concentration and depth instudied groundwater arranged by location, depth, and type of wellsbrine) affecting the Kill Devil Hills well field could haveimplications for predicting future salinity trends. The lowsalinity groundwater in shallow wells at Kill Devil Hills(1–310, Albatross, and Ocean Bay; Table 2) exhibits highNa/Cl ( 1) and B/Cl ( 2 10–3), low Mg/Cl and Ca/Clratios, and low δ11B (23–29‰) relative to the compositionof modern seawater (δ11B 39‰; B/Cl 8 10–4; Figs. 6and 7). In contrast, the deep groundwater is saline and hassome geochemical properties that resemble seawater (e.g.,Na/Cl close to the seawater value of 0.86; Br/Cl close tothe seawater value of 1.5 10–3; Table 2). This composition suggests reverse base-exchange reactions, in whichCa- and Mg-bearing recharge interacts with marine claysin which the exchange sites are occupied by Na due toprior contact with seawater. In a reverse base-exchangereaction, Ca2 and Mg2 are lost from the groundwater tothe solids in exchange for Na , resulting in Na enrichment5a 1015 20Depth to water (m) 1 5101 610251 310Production well 119901995200020052010Yearb 750010000 500002500Chloride (mg/L) 19901995200020052010YearFig. 4 Historical water data for production well 1 and adjacent monitoring wells (1-310, 1-510, 1-610) from 1989 through 2009: a waterlevel measurements; b chloride concentrations. Data provided by Dare County Water Department. Trend lines calculated using LOWESSmethod in which smoothing is controlled by the nearest 20% of data points (R Development Core Team 2009)Hydrogeology Journal (2011) 19: 981–994DOI 10.1007/s10040-011-0738-x
988b5 1a0 5 10δ2H 4.8 δ18O 0.2‰ 20 15δ2H (‰) 3 5 30 25 4δ18O (‰) 2Mainland & Roanoke IslandShallow monitoring wellsProduction wellsDeep monitoring wells0200040006000800010000 5 4 3 2 10δ18O (‰)Cl (mg/L)Fig. 5 a Oxygen and b hydrogen isotope ratios in groundwater plotted along possible mixing trends. In a the dashed line representshypothetical mixing of fresh locally recharged groundwater (20 mg/L Cl, δ2H 20‰, δ18O 4.5‰) with a seawater-like salinegroundwater ( 19,000 mg/L Cl, δ2H and δ18O 0‰). In b the dashed line represents a linear regression of the δ2H and δ18O data. Note they-intercept value of δ2H 0‰, indicating a mixing relationship with seawater-like saline water rather than meteoric water that would exhibitdeuterium excessin the residual groundwater (Chapelle and Knobel1983; Knobel et al. 1998). Reverse base-exchangereactions can also explain the relatively high B/Cland low δ11B values (Fig. 7) that are consistent withthe composition expected from boron desorption fromclay minerals (Spivack et al. 1987). In contrast, indirect base-exchange reactions that characterize seawater intrusion zones, boron is retained by adsorptiononto clay minerals, resulting in high δ11B and low B/Cl in the residual groundwater relative to seawatervalues (Jones et al. 1999; Vengosh and Spivack 1999;Vengosh 2003). Although Ca/Cl and Sr/Cl ratiosexceed seawater values in the deep saline groundwater,as is seen in seawater intrusion zones (Nadler et al.1980; Vengosh et al. 2002; Andersen et al. 2005;Sivan et al 2005), Mg/Cl is significantly depletedrelative to the seawater ratio. Together, these ratiosimply that mixing and divalent-for-monovalentexchange cations cannot completely explain the Caand Mg concentrations in the groundwater system;Table 2 Ion (mol/mol) and isotopic ratios of groundwater and reverse osmosis plant waterSample nameNa/ClMg/ClMainland and Roanoke Island monitoring wellsJ7K71.931.52J7K51.240.032J3O32.080.12Kill Devil Hills coastal monitoring wellsOcean Bay2.350.026Albatross1.280.054Kill Devil Hills well field1-3101.760.024Production well 11.010.0711-5100.940.0911-6100.910.083Production well 21.010.072Production well 70.970.081Production well 110.970.071Reverse osmosis 96abCa/ClSr/Cl( 10–4)SO42–/ClB/Cl( rom samples collected 20–21 Nov 2008Vengosh and Spivack (1999)Hydrogeology Journal (2011) 19: 981–994DOI 10.1007/s10040-011-0738-x
250150Na 100Cl (mM)2002503002002503000.200.0000150.050.10Sr (mM)43210Ca (mM)1550.15620150Cl (mM)7Cl (mM)Mg (mM)100624SO42 (mM)0.30.20.1Br (mM)0.4Mainland & Roanoke IslandShallow monitoring wellsProduction wellsDeep monitoring wells20080.5989050100150200250300050100Cl (mM)150200250300050100Cl (mM)150Cl (mM)Fig. 6 Relationships between chloride and major ion concentrations in the investigated groundwater. Dashed lines represent hypotheticaldilution of seawater. Note that the compositions of the production wells show a mixing relationship between deep and shallow groundwaterrepresenting about 20–25% input of deep, saline waterof modified and apparently old seawater with localrecharge, and not modern seawater intrusion. Thismixing relationship is also modified by reverse baseexchange reactions and boron desorption in theshallow, fresh portion of the aquifer. Mixing with oldseawater at depth is consistent with previous studies ofthe Atlantic Coastal Plain (see section Hydrogeologicsetting), and can also explain the relatively low rate of3.0some other phenomenon such as carbonate equilibriummay be necessary. The highest Ca/Cl and Mg/Cl ratiosin the study area are seen in shallow wells inland fromthe Kill Devil Hills well field (J3O3 and J7K7;Table 2) that are less affected by reverse cationexchange due to the freshening impact of recharge.Overall, ion ratios and δ11B values suggest that thesalinity of the pumping wells is derived from mixing4035 Seawater2530δ11B (‰)2.01.51.00.5B (mg/L)2.5Mainland & Roanoke IslandShallow monitoring wellsProduction wellsDeep monitoring wellsb200.0a0200040006000Cl (mg/L)80001000010 410 310 210 1B /Cl (mol/mol log scale)Fig. 7 Relationship between a boron and chloride contents and b δ11B and B/Cl ratios (log scale) in the investigated groundwater. Notethat studied groundwater samples have higher B/Cl and lower δ11B values relative to seawaterHydrogeol
local groundwater resources. In Dare County, North Carolina, which includes the barrier island areas that are the setting for this study (Fig. 1), population grew from 23,000 to 34,000from 1991 to 2009, a 48% increase (North Carolina, Office of State Budget and Management, State Demographics Branch 2010). In addition, Dare County has