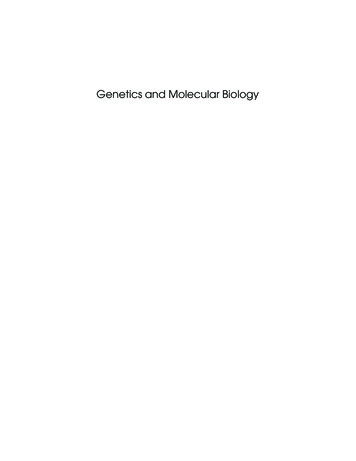
Transcription
Genetics and Molecular Biology
Genetics andMolecular BiologyS E C O N DE D I T I O NRobert SchleifDepartment of BiologyThe Johns Hopkins UniversityBaltimore, MarylandThe Johns Hopkins University Press Baltimore and London
1986 by Addison-Wesley Publishing Company 1993 by Robert SchleifAll rights reservedPrinted in the United States of America on acid-free paperThe Johns Hopkins University Press2715 North Charles StreetBaltimore, Maryland 21218-4319The Johns Hopkins Press Ltd., LondonLibrary of Congress Cataloging-in-Publication DataSchleif, Robert F.Genetics and molecular biology / by Robert Schleif.—2nd ed.p.cm.Includes bibliographical references and index.ISBN 0-8018-4673-0 (acid-free paper).—ISBN 0-8018-4674-9 (pbk : acid-freepaper)1. Molecular genetics. I. TitleQH442.S34 1993The catalog record for this book is available from the British Library.
PrefaceThis book evolved from a course in molecular biology which I have beenteaching primarily to graduate students for the past twenty years.Because the subject is now mature, it is possible to present the materialby covering the principles and encouraging students to learn how toapply them. Such an approach is particularly efficient as the subject ofmolecular genetics now is far too advanced, large, and complex formuch value to come from attempting to cover the material in anencyclopedia-like fashion or teaching the definitions of the relevantwords in a dictionary-like approach. Only the core of molecular geneticscan be covered by the present approach. Most of the remainder of thevast subject however, is a logical extension of the ideas and principlespresented here. One consequence of the principles and analysis approach taken here is that the material is not easy. Thinking and learningto reason from the fundamentals require serious effort, but ultimately,are more efficient and more rewarding than mere memorization.An auxiliary objective of this presentation is to help students developan appreciation for elegant and beautiful experiments. A substantialnumber of such experiments are explained in the text, and the citedpapers contain many more.The book contains three types of information. The main part of eachchapter is the text. Following each chapter are references and problems.References are arranged by topic, and one topic is “Suggested Readings”. The additional references cited permit a student or researcher tofind many of the fundamental papers on a topic. Some of these are ontopics not directly covered in the text. Because solving problems helpsfocus one’s attention and stimulates understanding, many thought-provoking problems or paradoxes are provided. Some of these require useof material in addition to the text. Solutions are provided to about halfof the problems.v
vi PrefaceAlthough the ideal preparation for taking the course and using thebook would be the completion of preliminary courses in biochemistry,molecular biology, cell biology, and physical chemistry, few studentshave such a background. Most commonly, only one or two of theabove-mentioned courses have been taken, with some students comingfrom a more physical or chemical background, and other studentscoming from a more biological background.My course consists of two lectures and one discussion session perweek, with most chapters being covered in one lecture. The lecturesoften summarize material of a chapter and then discuss in depth arecent paper that extends the material of the chapter. Additional readings of original research papers are an important part of the course forgraduate students, and typically such two papers are assigned perlecture. Normally, two problems from the ends of the chapters areassigned per lecture.Many of the ideas presented in the book have been sharpened by myfrequent discussions with Pieter Wensink, and I thank him for this. Ithank my editors, James Funston for guidance on the first edition andYale Altman and Richard O’Grady for ensuring the viability of thesecond edition. I also thank members of my laboratory and the followingwho read and commented on portions of the manuscript: KarenBeemon, Howard Berg, Don Brown, Victor Corces, Jeff Corden, DavidDraper, Mike Edidin, Bert Ely, Richard Gourse, Ed Hedgecock, RogerHendrix, Jay Hirsh, Andy Hoyt, Amar Klar, Ed Lattman, RogerMcMacken, Howard Nash, and Peter Privalov.
Contents1 An Overview of Cell Structure and FunctionCell’s Need for Immense Amounts of InformationRudiments of Prokaryotic Cell StructureRudiments of Eukaryotic Cell StructurePacking DNA into CellsMoving Molecules into or out of CellsDiffusion within the Small Volume of a CellExponentially Growing PopulationsComposition Change in Growing CellsAge Distribution in Populations of Growing CellsProblemsReferences2 Nucleic Acid and Chromosome StructureThe Regular Backbone Of DNAGrooves in DNA and Helical Forms of DNADissociation and Reassociation of Base-paired StrandsReading Sequence Without Dissociating StrandsElectrophoretic Fragment SeparationBent DNA SequencesMeasurement of Helical PitchTopological Considerations in DNA StructureGenerating DNA with Superhelical TurnsMeasuring Superhelical TurnsDetermining Lk, Tw, and Wr in Hypothetical StructuresAltering Linking NumberBiological Significance of Superhelical 9vii
viii ContentsThe Linking Number Paradox of NucleosomesGeneral Chromosome StructureSouthern Transfers to Locate Nucleosomes on GenesARS Elements, Centromeres, and TelomeresProblemsReferences3 DNA Synthesis40414143444753A. Enzymology54Proofreading, Okazaki Fragments, and DNA LigaseDetection and Basic Properties of DNA PolymerasesIn vitro DNA ReplicationError and Damage Correction54576062B. Physiological Aspects66DNA Replication Areas In ChromosomesBidirectional Replication from E. coli OriginsThe DNA Elongation RateConstancy of the E. coli DNA Elongation RateRegulating InitiationsGel Electrophoresis Assay of Eukaryotic Replication OriginsHow Fast Could DNA Be Replicated?ProblemsReferences6667697172747678794 RNA Polymerase and RNA InitiationMeasuring the Activity of RNA PolymeraseConcentration of Free RNA Polymerase in CellsThe RNA Polymerase in Escherichia coliThree RNA Polymerases in Eukaryotic CellsMultiple but Related Subunits in PolymerasesMultiple Sigma SubunitsThe Structure of PromotersEnhancersEnhancer-Binding ProteinsDNA Looping in Regulating Promoter ActivitiesSteps of the Initiation ProcessMeasurement of Binding and Initiation RatesRelating Abortive Initiations to Binding and InitiatingRoles of Auxiliary Transcription FactorsMelted DNA Under RNA PolymeraseProblemsReferences5 Transcription, Termination, and RNA ProcessingPolymerase Elongation 19119
Contents ixTranscription Termination at Specific SitesTerminationProcessing Prokaryotic RNAs After SynthesisS1 Mapping to Locate 5’ and 3’ Ends of TranscriptsCaps, Splices, Edits, and Poly-A Tails on Eukaryotic RNAsThe Discovery and Assay of RNA SplicingInvolvement of the U1 snRNP Particle in SplicingSplicing Reactions and ComplexesThe Discovery of Self-Splicing RNAsA Common Mechanism for Splicing ReactionsOther RNA Processing ReactionsProblemsReferences6 Protein StructureThe Amino AcidsThe Peptide BondElectrostatic Forces that Determine Protein StructureHydrogen Bonds and the Chelate EffectHydrophobic ForcesThermodynamic Considerations of Protein StructureStructures within ProteinsThe Alpha Helix, Beta Sheet, and Beta TurnCalculation of Protein Tertiary StructureSecondary Structure PredictionsStructures of DNA-Binding ProteinsSalt Effects on Protein-DNA InteractionsLocating Specific Residue-Base InteractionsProblemsReferences7 Protein . Chemical Aspects184Activation of Amino Acids During Protein SynthesisFidelity of AminoacylationHow Synthetases Identify the Correct tRNA MoleculeDecoding the MessageBase Pairing between Ribosomal RNA and MessengerExperimental Support for the Shine-Dalgarno HypothesisEukaryotic Translation and the First AUGTricking the Translation Machinery into InitiatingProtein ElongationPeptide Bond FormationTranslocationTermination, Nonsense, and SuppressionChaperones and Catalyzed Protein Folding184185187188191192194195197198198199202
x ContentsResolution of a Paradox202B. Physiological Aspects203Messenger InstabilityProtein Elongation RatesDirecting Proteins to Specific Cellular SitesVerifying the Signal Peptide ModelThe Signal Recognition Particle and TranslocationExpectations for Ribosome RegulationProportionality of Ribosome Levels and Growth RatesRegulation of Ribosome SynthesisBalancing Synthesis of Ribosomal 142162182208 GeneticsMutationsPoint Mutations, Deletions, Insertions, and DamageClassical Genetics of ChromosomesComplementation, Cis, Trans, Dominant, and RecessiveMechanism of a trans Dominant Negative MutationGenetic RecombinationMapping by Recombination FrequenciesMapping by DeletionsHeteroduplexes and Genetic RecombinationBranch Migration and IsomerizationElements of Recombination in E. coli, RecA, RecBCD, and ChiGenetic SystemsGrowing Cells for Genetics ExperimentsTesting Purified Cultures, ScoringIsolating Auxotrophs, Use of Mutagens and Replica PlatingGenetic SelectionsMapping with Generalized Transducing PhagePrinciples of Bacterial SexElements of Yeast GeneticsElements of Drosophila GeneticsIsolating Mutations in Muscle or Nerve in DrosophilaFate Mapping and Study of Tissue-Specific Gene ExpressionProblemsReferences9 Genetic Engineering and Recombinant DNAThe Isolation of DNAThe Biology of Restriction EnzymesCutting DNA with Restriction EnzymesIsolation of DNA 5246247248250251253254255256257261265266268271272
Contents xiJoining DNA FragmentsVectors: Selection and Autonomous DNA ReplicationPlasmid VectorsA Phage Vector for BacteriaVectors for Higher CellsPutting DNA Back into CellsCloning from RNAPlaque and Colony Hybridization for Clone IdentificationWalking Along a Chromosome to Clone a GeneArrest of Translation to Assay for DNA of a GeneChemical DNA SequencingEnzymatic DNA SequencingProblemsReferences10 Advanced Genetic EngineeringFinding Clones from a Known Amino Acid SequenceFinding Clones Using Antibodies Against a ProteinSouthern, Northern, and Western TransfersPolymerase Chain ReactionIsolation of Rare Sequences Utilizing PCRPhysical and Genetic Maps of ChromosomesChromosome MappingDNA Fingerprinting—ForensicsMegabase SequencingFootprinting, Premodification and Missing Contact ProbingAntisense RNA: Selective Gene InactivationHypersynthesis of ProteinsAltering Cloned DNA by in vitro MutagenesisMutagenesis with Chemically Synthesized DNAProblemsReferences11 Repression and the lac OperonBackground of the lac OperonThe Role of Inducer Analogs in the Study of the lac OperonProving lac Repressor is a ProteinAn Assay for lac RepressorThe Difficulty of Detecting Wild-Type lac RepressorDetection and Purification of lac RepressorRepressor Binds to DNA: The Operator is DNAThe Migration Retardation Assay and DNA LoopingThe Isolation and Structure of OperatorIn vivo Affinity of Repressor for OperatorThe DNA-binding Domain of lac RepressorA Mechanism for 25331332334335336338340341343344346346348
xii ContentsProblemsReferences12 Induction, Repression, and the araBAD OperonThe Sugar Arabinose and Arabinose MetabolismGenetics of the Arabinose SystemDetection and Isolation of AraC ProteinRepression by AraCRegulating AraC SynthesisBinding Sites of the ara Regulatory ProteinsDNA Looping and Repression of araBADIn vivo Footprinting Demonstration of LoopingHow AraC Protein Loops and UnloopsWhy Looping is Biologically SensibleWhy Positive Regulators are a Good IdeaProblemsReferences13 Attenuation and the trp OperonThe Aromatic Amino Acid Synthetic Pathway and its RegulationRapid Induction Capabilities of the trp OperonThe Serendipitous Discovery of trp Enzyme HypersynthesisEarly Explorations of the Hypersynthesistrp Multiple Secondary Structures in trp Leader RNACoupling Translation to TerminationRNA Secondary Structure and the Attenuation MechanismOther Attenuated Systems: Operons, Bacillus subtilis and HIVProblemsReferences14 Lambda Phage Genes and Regulatory 6377379385386388390392396397399400402404409A. The Structure and Biology of Lambda410The Physical Structure of LambdaThe Genetic Structure of LambdaLysogeny and ImmunityLambda’s Relatives and Lambda Hybrids410411413414B. Chronology of a Lytic Infective Cycle415Lambda Adsorption to CellsEarly Transcription of Genes N and CroN Protein and Antitermination of Early Gene TranscriptionThe Role of Cro ProteinInitiating DNA Synthesis with the O and P ProteinsProteins Kil, γ, β, and ExoQ Protein and Late Proten SynthesisLysis415416417418418419420421
Contents xiiiC. The Lysogenic Infective Cycle and Induction of Lysogens422Chronology of Becoming a LysogenSite for Cro Repression and CI ActivationCooperativity in Repressor Binding and its MeasurementThe Need for and the Realization of Hair-Trigger InductionInduction from the Lysogenic StateEntropy, a Basis for Lambda Repressor 343515 Xenopus 5S RNA SynthesisBiology of 5S RNA Synthesis in XenopusIn vitro 5S RNA SynthesisTFIIIA Binding to the Middle of its Gene as Well as to RNASwitching from Oocyte to Somatic 5S SynthesisStructure and Function of TFIIIAProblemsReferences16 Regulation of Mating Type in YeastThe Yeast Cell CycleMating Type Conversion in Saccharomyces cerevisiaeCloning the Mating Type Loci in YeastTransfer of Mating Type Gene Copies to an Expression SiteStructure of the Mating Type LociThe Expression and Recombination ParadoxesSilencing HML and HMRIsolation of α2 Proteinα2 and MCM1Sterile Mutants, Membrane Receptors and G FactorsDNA Cleavage at the MAT LocusDNA Strand Inheritance and Switching in Fission YeastProblemsReferences17 Genes Regulating DevelopmentGeneral Considerations on SignalingOutline of Early Drosophila DevelopmentClassical EmbryologyUsing Genetics to Begin Study of Developmental SystemsCloning Developmental GenesEnhancer Traps for Detecting and Cloning Developmental GenesExpression Patterns of Developmental GenesSimilarities Among Developmental GenesOverall Model of Drosophila Early 7488491491
xiv ContentsProblemsReferences18 Lambda Phage Integration and ExcisionMapping Integrated LambdaSimultaneous Deletion of Chromosomal and Lambda DNADNA Heteroduplexes Prove that Lambda IntegratesGene Order Permutation and the Campbell ModelIsolation of Integration-Defective MutantsIsolation of Excision-Deficient MutantsProperties of the int and xis Gene ProductsIncorrect Excision and gal and bio Transducing PhageTransducing Phage Carrying Genes Other than gal and bioUse of Transducing Phage to Study Integration and ExcisionThe Double att Phage, att2Demonstrating Xis is UnstableInhibition By a Downstream ElementIn vitro Assay of Integration and ExcisionHost Proteins Involved in Integration and ExcisionStructure of the att RegionsStructure of the IntasomeHolliday Structures and Branch Migration in IntegrationProblemsReferences19 Transposable Genetic ElementsIS Elements in BacteriaStructure and Properties of IS ElementsDiscovery of Tn ElementsStructure and Properties of Tn ElementsInverting DNA Segments by Recombination, Flagellin SynthesisMu Phage As a Giant Transposable ElementAn Invertible Segment of Mu PhageIn vitro Transposition, Threading or Global Topology?Hopping by Tn10Retrotransposons in Higher CellsAn RNA Transposition IntermediateP Elements and TransformationP Element Hopping by Chromosome RescueProblemsReferences20 Generating Genetic Diversity: AntibodiesThe Basic Adaptive Immune ResponseTelling the Difference Between Foreign and SelfThe Number of Different Antibodies 4545547550552553555557558563563565566
Contents xvMyelomas and Monoclonal AntibodiesThe Structure of AntibodiesMany Copies of V Genes and Only a Few C GenesThe J RegionsThe D Regions in H ChainsInduced Mutations and Antibody DiversityClass Switching of Heavy ChainsEnhancers and Expression of Immunoglobulin GenesThe AIDS VirusEngineering Antibody Synthesis in BacteriaAssaying for Sequence Requirements of Gene RearrangementsCloning the RecombinaseProblemsReferences21 Biological Assembly, Ribosomes and Lambda A. Ribosome Assembly592RNAse and RibosomesThe Global Structure of RibosomesAssembly of RibosomesExperiments with in vitro Ribosome AssemblyDetermining Details of Local Ribosomal Structure592593595597599B. Lambda Phage Assembly601General AspectsThe Geometry of CapsidsThe Structure of the Lambda ParticleThe Head Assembly Sequence and Host ProteinsPackaging the DNA and Formation of the cos EndsFormation of the TailIn vitro 061322 ChemotaxisAssaying ChemotaxisFundamental Properties of ChemotaxisGenetics of Motility and ChemotaxisHow Cells SwimThe Mechanism of ChemotaxisThe Energy for ChemotaxisAdaptationMethylation and AdaptationPhosphorylation and the Rapid 632633635637
xvi Contents23 Oncogenesis, Molecular AspectsBacterially Induced Tumors in PlantsTransformation and Oncogenesis by Damaging the ChromosomeIdentifying a Nucleotide Change Causing CancerRetroviruses and CancerCellular Counterparts of Retroviral OncogenesIdentification of the src and sis Gene ProductsDNA Tumor VirusesRecessive Oncogenic Mutations, Tumor SuppressorsThe ras-fos-jun PathwayDirections for Future Research in Molecular 58660661661663Hints and Solutions to Odd-Numbered Problems667Index685
An Overview of CellStructure and Function1In this book we will be concerned with the basics of the macromolecularinteractions that affect cellular processes. The basic tools for suchstudies are genetics, chemistry, and physics. For the most part, we willbe concerned with understanding processes that occur within cells, suchas DNA synthesis, protein synthesis, and regulation of gene activity. Theinitial studies of these processes utilize whole cells. These normally arefollowed by deeper biochemical and biophysical studies of individualcomponents. Before beginning the main topics we should take time foran overview of cell structure and function. At the same time we shoulddevelop our intuitions about the time and distance scales relevant to themolecules and cells we will study.Many of the experiments discussed in this book were done with thebacterium Escherichia coli, the yeast Saccharomyces cerevisiae, and thefruit fly Drosophila melanogaster. Each of these organisms possessesunique characteristics making it particularly suitable for study. In fact,most of the research in molecular biology has been confined to thesethree organisms. The earliest and most extensive work has been donewith Escherichia coli. The growth of this oranism is rapid and inexpensive, and many of the most fundamental problems in biology aredisplayed by systems utilized by this bacterium. These problems aretherefore most efficiently studied there. The eukaryotic organisms arenecessary for study of phenomena not observed in bacteria, but parallelstudies on other bacteria and higher cells have revealed that the basicprinciples of cell operation are the same for all cell types.1
2 An Overview of Cell Structure and FunctionCell’s Need for Immense Amounts of InformationCells face enormous problems in growing. We can develop some idea ofthe situation by considering a totally self-sufficient toolmaking shop. Ifwe provide the shop with coal for energy and crude ores, analogous toa cell’s nutrient medium, then a very large collection of machines andtools is necessary merely to manufacture each of the parts present inthe shop. Still greater complexity would be added if we required thatthe shop be totally self-regulating and that each machine be self-assembling. Cells face and solve these types of problems. In addition, each ofthe chemical reactions necessary for growth of cells is carried out in anaqueous environment at near neutral pH. These are conditions thatwould cripple ordinary chemists.By the tool shop analogy, we expect cells to utilize large numbers of“parts,” and, also by analogy to factories, we expect each of these partsto be generated by a specialized machine devoted to production of justone type of part. Indeed, biochemists’ studies of metabolic pathwayshave revealed that an E. coli cell contains about 1,000 types of parts, orsmall molecules, and that each is generated by a specialized machine,an enzyme. The information required to specify the structure of evenone machine is immense, a fact made apparent by trying to describe anobject without pictures and drawings. Thus, it is reasonable, and indeedit has been found that cells function with truly immense amounts ofinformation.DNA is the cell’s library in which information is stored in its sequenceof nucleotides. Evolution has built into this library the informationnecessary for cells’ growth and division. Because of the great value ofthe DNA library, it is natural that it be carefully protected and preserved.Except for some of the simplest viruses, cells keep duplicates of theinformation by using a pair of self-complementary DNA strands. Eachstrand contains a complete copy of the information, and chemical orphysical damage to one strand is recognized by special enzymes and isrepaired by making use of information contained on the oppositestrand. More complex cells further preserve their information by possessing duplicate DNA duplexes.Much of the recent activity in molecular biology can be understoodin terms of the cell’s library. This library contains the informationnecessary to construct the different cellular machines. Clearly, such alibrary contains far too much information for the cell to use at any onetime. Therefore mechanisms have developed to recognize the need forparticular portions, “books,” of the information and read this out of thelibrary in the form of usable copies. In cellular terms, this is theregulation of gene activity.Rudiments of Prokaryotic Cell StructureA typical prokaryote, E. coli, is a rod capped with hemispheres (Fig. 1.1).It is 1–3 µ (10-4 cm 1 µ 104 Å) long and 0.75 µ in diameter. Such a
Rudiments of Prokaryotic Cell Structure 3RibosomesNuclearregionCellenvelope0.75µFigure 1.1 The dimensions of atypical E. coli cell.2µcell contains about 2 10-13 g of protein, 2 10-14 g of RNA that is mostlyribosomal RNA, and 6 10-15 g of DNA.The cell envelope consists of three parts, an inner and outer membrane and an intervening peptidoglycan layer (Fig. 1.2). The outersurface of the outer membrane is largely lipopolysaccharides. These areattached to lipids in the outer half of the outer membrane. The polysaccharides protect the outer membrane from detergent-like moleculesfound in our digestive tract.outer membrane The outer membrane alsoconsists of matrix proteins that form pores small enough to exclude thedetergent-like bile salts, but large enough to permit passage of smallmolecules and phospholipids.Figure 1.2 Schematic drawing of the structure of the envelope of an E. nLipidsOutermembranePeptidoglycanor cell wallPeriplasmicspaceInnermembranePeriplasmic proteinPhospholipidsProteinsPhospholipid
4 An Overview of Cell Structure and 0-60 units in E. coliMN-acetylmuramic acidCH2 OHCH2 OHOH HOH H HOHHNHCCH 3OH HOOHHOHHOCCOOHCH3NHCOCH3(b)Figure 1.3 Structure of the cell wall showing the alternating N-acetylglucosamine N-acetylmuramic acid units. Each N-acetylmuramic acid possessesa peptide, but only a few are crosslinked in E. coli.The major shape-determining factor of cells is the peptidoglycanlayer or cell wall (Fig. 1.3). It lies beneath the outer membrane and is asingle molecule containing many polysaccharide chains crosslinked byshort peptides (Fig. 1.4). The outer membrane is attached to the peptidoglycan layer by about 106 lipoprotein molecules. The protein end ofeach of these is covalently attached to the diaminopimelic acid in thepeptidoglycan. The lipid end is buried in the outer membrane.The innermost of the three cell envelope layers is the inner orcytoplasmic membrane. It consists of many proteins embedded in aphospholipid bilayer. The space between the inner membrane and theouter membrane that contains the peptidoglycan layer is known as theperiplasmic space. The cell wall and membranes contain about 20% ofthe cellular protein. After cell disruption by sonicating or grinding, mostof this protein is still contained in fragments of wall and membrane andcan be easily pelleted by low-speed centrifugation.The cytoplasm within the inner membrane is a protein solution atabout 200 mg/ml, about 20 times more concentrated than the usualcell-free extracts used in the laboratory. Some proteins in the cytoplasmmay constitute as little as 0.0001% by weight of the total cellular proteinwhereas others may be found at levels as high as 5%. In terms ofconcentrations, this is from 10-8 M to 2 10-4 M, and in a bacterial cellthis is from 10 to 200,000 molecules per cell. The concentrations ofmany of the proteins vary with growth conditions, and a current research area is the study of the cellular mechanisms responsible for thevariations.The majority of the more than 2,000 different types of proteins foundwithin a bacterial cell are located in the cytoplasm. One question yet to
Rudiments of Prokaryotic Cell Structure 5N-acetylmuramic acidOHOOCCHNHAcOOHHOOHOH CCH 3 CCNCCNHCH 3L-AlaCHD-GluN-acetylmuramic acidCH2(CH 2) 3CH 2OHCNCOHCH 3CNCHOHOCHmeso -DAPD-AlaN-acetylmuramic Figure 1.4 Structure of the peptide crosslinking N-acetylmuramic acid units.DAP is diaminopimelic acid.be answered about these proteins is how they manage to exist in the cellwithout adhering to each other and forming aggregates since polypeptides can easily bind to each other. Frequently when a bacterium isengineered for the over-synthesis of a foreign protein, amorphousprecipitates called inclusion bodies form in the cytoplasm. Sometimesthese result from delayed folding of the new protein, and occasionallythey are the result of chance coprecipitation of a bacterial protein andthe newly introduced protein. Similarly, one might also expect anoccasional mutation to inactivate simultaneously two apparently unrelated proteins by the coprecipitation of the mutated protein and someother protein into an inactive aggregate, and occasionally this doesoccur.The cell’s DNA and about 10,000 ribosomes also reside in the cytoplasm. The ribosomes consist of about one-third protein and two-thirdsRNA and are roughly spherical with a diameter of about 200 Å. The DNAin the cytoplasm is not surrounded by a nuclear membrane as it is inthe cells of higher organisms, but nonetheless it is usually confined toa portion of the cellular interior. In electron micrographs of cells, thehighly compacted DNA can be seen as a stringy mass occupying aboutone tenth of the interior volume, and the ribosomes appear as granulesuniformly scattered through the cytoplasm.
6 An Overview of Cell Structure and FunctionRudiments of Eukaryotic Cell StructureA typical eukaryotic cell is 10 µ in diameter, making its volume about1,000 times that of a bacterial cell. Like bacteria, eukaryotic cells containcell membranes, cytoplasmic proteins, DNA, and ribosomes, albeit ofsomewhat different structure from the corresponding prokaryotic elements (Fig. 1.5). Eukaryotic cells, however, possess many structuralfeatures that even more clearly distinguish them from prokaryotic cells.Within the eukaryotic cytoplasm are a number of structural proteinsthat form networks. Microtubules, actin, intermediate filaments, andthin filaments form four main categories of fibers found within eukaryotic cells. Fibers within the cell provide a rigid structural skeleton,participate in vesicle and chromosome movement, and participate inchanging the cell shape so that it can move. They also bind the majorityof the ribosomes.The DNA of eukaryotic cells does not freely mix with the cytoplasm,but is confined within a nuclear membrane. Normally only small proteins of molecular weight less than 20 to 40,000 can freely enter thenucleus through the nuclear membrane. Larger proteins and nuclearRNAs enter the nucleus through special nuclear pores. These are largestructures that actively transport proteins or RNAs into or out of thenucleus. In each cell cycle, the nuclear membrane dissociates, and thenlater reaggregates. The DNA itself is tightly complexed with a class ofproteins called histones, whose main function appears to be to help DNAretain a condensed state. When the cell divides, a special apparatuscalled the spindle, and consisting in part of microtubules, is necessaryto pull the chromosomes into the daughter cells.Eukaryotic cells also contain specialized organelles such as mitochondria, which perform oxidative phosphorylation to generate thecell’s needed chemical energy. In many respects mitochondria resemblebacteria and, in fact, appear to have evolved from bacteria. They containDNA, usually in the form of a circular chromosome like that of E. coliFigure 1.5 S c h e m a t i cdrawing of a µNuclearmembraneNucleusEndoplasmicreticulumGolgi ap
Printed in the United States of America on acid-free paper The Johns Hopkins University Press 2715 North Charles Street Baltimore, Maryland 21218-4319 The Johns Hopkins Press Ltd., London Library of Congress Cataloging-in-Publication Data Schleif, Robert F. Genetics and molec